Zeolite Synthesis and Preparation of Artifical Zeolite
Zeolite:
In 1756, Swedish geologist Axel Cronstedt (1722–1765) best known as the pioneer of nickel—begat the name "zeolite".
Zeolites are natural volcanic minerals that are mined in specific parts of the world. At the point when volcanoes eject, liquid magma and thick cinder(ash) blasts out. Since numerous volcanoes are situated on an island or close to a sea, this magma and cinder frequently streams into the ocean.
Heat a glass of water and you'll see steam ascend off it eventually as it goes to the boil. You absolutely don't anticipate that the same thing will happen in the event that you warm a stone unless it's an exceptional sort of rock called a zeolite, which traps water inside it. since it truly signifies "bubbling stone"; today, the term alludes to more than 200 unique minerals that have a wide range of fascinating uses, from water conditioners and feline litter to creature sustenance and modern impetuses.
Zeolites structure with various crystalline structures, which have vast open pores (here and there alluded to as cavities) in an exceptionally normal game plan and generally the same size as little particles. Zeolites are hydrated aluminosilicate minerals produced using interlinked tetrahedra of alumina (AlO4) and silica (SiO4). In more straightforward words, they're solids with a generally open, three-dimensional precious stone structure worked from the components aluminum, oxygen, and silicon, with soluble base or basic Earth metals, (for example, sodium, potassium, and magnesium) in addition to water atoms caught in the holes between them.
A large portion of the zeolites found in nature are thermally steady. Some transitional stages (meta stable) can not be found in nature, in light of the fact that their security changes into the more steady one in the wake of breathing easy or augmented warming time. In research facility one can tune for the most part system sort wanted for his application, in a large portion of the cases one can likewise tune the substance creation of the structure. The metastable zeolite development can be accomplished in the lab by extinguishing the union response. Be that as it may, a portion of the zeolites are there they are combined in the research facility as of late, for example, natrolite iin 2014. Aside from this the system structure will be verging on comparable for regular and manufactured zeolite of same topology, and as a consequence of it nature of XRD example. Later can be distinctive if one modifies the substance structure (shift in top positions)... and so forth...
One more distinction is there,, in lab on can blend the huge measure of required zeolite while nature has impediments so for mechanical applications one can get mass sum from pilot plants.
Important Uses:
1.100% safe and non-dangerous at any level: All hints of the mineral are totally dispensed with out of the body inside of 6 to 8 hours. It is protected to bring with most prescriptions (the length of they are not substantial metal based), but rather as dependably you ought to check with a therapeutic expert first. *
2. Uproots substantial metals and poisons: Micronized zeolite has the ideal sub-atomic structure for catching and expelling overwhelming metals from the body without evacuating sound particles and minerals. (as clarified in chart above). Numerous individuals report feeling expanded vitality, clarity and essentialness in the wake of evacuating overwhelming metal develop. *
3. Equalizations body's pH levels to a sound alkalinity: Whenever micronized zeolite is acquainted with the body, it cradles the framework towards marginally basic (pH 7.35 to 7.45), which is an ideal pH for the human body. Zeolite draws in and after that cradles overabundance protons which cause sharpness and along these lines can help numerous conditions from heartburn to Candida and joint inflammation.
4. Zeolite is anything but difficult to take: Most detoxification items are hard to take and numerous must be infused into the blood or directed, for example, a bowel purge. Zeolite works at the cell level, has no taste or scent and it can be tackled a void stomach. (you wont even know you are taking it)
1. INTRODUCTION
1.1. Background:
Drinking water is one of the basic needs of life and essential for survival. Still more than one billion people all over the world do not have ready access to an adequate and safe water supply and more than 800 million of those unsaved live in rural areas. In India, ground water is being used as raw water for 85% public water supply. Water supply varies widely in terms of region and country. In 1970s, of the approximately 2.5 billion people in developing world, only 38% has safe drinking water. At the beginning of the 1980s, water supply coverage was 75% in urban areas and 46% in rural areas. In developing countries, 75% of the population had access to water supply. So they are always prone to loss of their lives or cost a big toll to save themselves from the occurrence of different water-borne disease.
1.2.Justification:
Water contamination due to pathogenic agents, chemicals, heavy metals, pesticides, water disinfectants, and thereby product as a consequence of industrial and agricultural activities leaching from soil, rocks, and atmospheric deposition and other human activities has become a hazard to human health in several regions of world.
Most of the water resources should be treated for purification before consumption. In some countries, groundwater is the main safe drinking water resource. In some cases, the resource does not satisfy to the desirable levels regarding their chemical properties, such as hardness, nitrate contamination, heavy metals, soluble iron, etc. Among them, water hardness can appear problematic in some cases; it can also be considered as an important aesthetic parameter.
However, because public acceptance of hardness differs remarkably according to local conditions, a maximum acceptable level has not been defined. In general, water supplies with total hardness higher than 300 mg/L can be tolerated by consumers but are considered as poor resources; while values higher than 600 mg/L are not acceptable for most of the domestic consumptions. Hardness in water refers to existing divalent ions, such as iron, manganese, calcium and magnesium. Among them, calcium and magnesium are known as the dominant species for water hardening.
Although it has been shown that water hardness doesn’t have serious health impact, it has been demonstrated that hard water is responsible for the formation of deposits in boiler and household facilities, as well as diverse influence on cleaning performances of detergents. Formation of precipitates may cause a decrease of heat transfer in boiler, a decrease of fluid rate, bursting of water pipe line, the formation of stain in dishes and clothes. Furthermore, high concentrations of Magnesium in drinking water may induce a bitter taste. In water purification and treatment plant, lime and soda ash are used for the removal of hardness. One of the main drawbacks of this process is the resulting waste, namely the large amount of liquid sludge produced, as well as the need for re-carbonation of the softened water. In addition, the use of additional chemicals to prevent sludge production is restricted and hence in most cases water hardness species cannot be fully removed. Ion exchange processes have been considered as an alternative approach which is commercially in use worldwide. However, high power consumption and periodical regeneration of saturated ion-exchanger resins are needed. Potential hazards of the effluent solutions resulting from the regeneration process should also be taken into account.
Recently, various methods including electrodeionization process, electro membrane processes, capacitive deionization, membrane and fluidized pellet reactor, ion exchange process and adsorption have been studied for the removal of a wide variety of ionic and molecular species from various water streams, including those responsible for hardness (e.g. Ca2+ and Mg2+ cations). Amongst the developed processes, adsorption has been widely studied for the uptake of various ionic and molecular species form water. Although the efficiency of activated carbon (AC) as an adsorbent, it cost remains its main drawback.
Hence, finding inexpensive and efficient adsorbents remains a topical matter to water treatment industries. Naturally occurring Zeolite have several applications in agriculture, water treatment, aquaculture, manufacturing of paper, gas adsorption and as a catalyst. Large quantities of Zeolite have been synthesized and incorporated as a part of detergents. Fly Ash has been identified for its activity similar to Zeolite and investigations were carried out further to Zeolite character of fly ash.
Zeolite have been synthesized by hydrothermal crystallization of silica gel, NaOH and Al salts. The high charge present in Zeolite is tetrahedral co-ordinates of Al which is normally takes place under high pH and temperature.
As Fly Ash contain mixed silicate of sodium and aluminium but depends on the coal from which fly ash have been generated and temperatures through which it has been passed. In the present study fly ash and alkali (Na2CO3) is taken in different ratios and thermal treatment is carried out in furnace at different temperatures 300, 400, 500, 600, 7000 C. A part of Na2CO3 attaches to fly ash enhancing Si: Na ratio. Thus formed fly ash is expected to be of sodium-alumino-silicate with replaceable charges. Exact structural elucidation is a complex procedure but Zeolite character can be quantified by measuring cation exchange capacity (CEC), which is done in present study by removal of hardness of water.
CEC values are increased with alkali and processing temperature up to certain values followed by a decrease. CEC of the values increases up to 60 minute and attain constant activity indicating maturity of product. Regeneration of each sample was carried out thrice and its values are found reduce its activity for each subsequent regeneration. However, its activity ceases to exist after three regenerations.
Zeolite is obtained out of the process is of very small particle size in the size range of less than 1 micron. Zeolite in powder form is difficult to handle, it is a major drawback in all these processes where Zeolites involved. This precludes their use in future applications such as packed bed in columns for waste water treatment. To overcome this problem, Zeolite material is subjected to size enlargement with sufficient porosity. It is made into pellets preventing it from drastic change of property.
In the present study, an attempt was made to find the efficiency of various available adsorbents in comparison with a newly prepared adsorbent in laboratory.
1.3.Objectives:
The main objectives of the study are given below: :
Ø To find the adsorptive capacity of LPZ,
Ø To find the effective time for adsorption,
Ø To determine the most cost effective adsorbent,
Ø To compare the relative merits and demerits of various adsorbents.
1.4. Limitations Of The Project:
.
All studies have limitations. However, it is important that you restrict the discussion to limitations related to the research problem under investigation. For example, if a meta-analysis of existing literature is not a stated purpose of research, it should not be discussed as a limitation. Do not apologize for not addressing issues that did not promise to investigate in Paper
Here are examples of limitations may need to describe and to discuss how they possibly impacted your findings.
Possible Methodological Limitations
The number of the units of analysis you use in your study is dictated by the type of research problem you are investigating. Note that, if sample size is too small, it will be difficult to find significant relationships from the data, as statistical tests normally require a larger sample size to ensure a representative distribution of the population and to be considered representative of groups of people to whom results will be generalized or transferred.
Lack of available and/or reliable data -- a lack of data or of reliable data will likely require to limit the scope of analysis, the size of your sample, or it can be a significant obstacle in finding a trend and a meaningful relationship. Need to not only describe these limitations but to offer reasons why you believe data is missing or is unreliable.
Lack of prior research studies on the topic -- citing prior research studies forms the basis of your literature review and helps lay a foundation for understanding the research problem you are investigating. Depending on the currency or scope of your research topic, there may be little, if any, prior research on topic. Note that this limitiation can serve as an important opportunity to describe the need for further research.
Measure used to collect the data -- sometimes it is the case that, after completing your interpretation of the findings, you discover that the way in which you gathered data inhibited your ability to conduct a thorough analysis of the results.
However, self-reported data contain several potential sources of bias that should be noted as limitations: (1) selective memory (remembering or not remembering experiences or events that occurred at some point in the past); (2) telescoping [recalling events that occurred at one time as if they occurred at another time]; (3) attribution [the act of attributing positive events and outcomes to one's own agency but attributing negative events and outcomes to external forces]; and, (4) exaggeration [the act of representing outcomes or embelishing events as more significant than is actually suggested from other data].
1.4. Organization Of Documentation:
Five chapters and an appendix make up the thesis. The introduction constitutes the first chapter and gives a background of the presence of hardness ions in the water and methods currently used their mitigation.
A summary of the relevant literature reviewed in the study is discussed in Chapter two; this chapter provides the necessary information on the success of different types of adsorbents, particularly inexpensive adsorbents used in the removal of hardness ions from water.
Chapter three provides a detailed description of the materials and methods used in the research. The types of adsorbents used to describe the adsorption of the hardness ions on to the adsorbent are described here.
Chapter four provides the results of the study as well as a comprehensive discussion of the research findings. This chapter compares and contracts the findings of this research with those of other researchers and provides explanations.
The thesis is finally concluded with Chapter five-Conclusions and Future scope, this chapter briefly states the conclusions drawn from the research and makes recommendations for future work based on the success and challenges from the present work. An Appendix of some of the tables produced from the research is provided to complete the write up.
2.LITERATURE REVIEW
Several works are reported in literature on Zeolites and hardness removed by adsorption. Extensive has been collected in the preparation of Fly Ash and hardness removed by various adsorbents. Works on hydrothermal treatment are dominant on testing domain, on isotherm study have been found in literature. The literature pertaining to Zeolite, adsorption and some other relevant works are presented in the following.
Suleyman A. Muyibi et al (1995)22., posed in his project that softening hard water by Moringaoleifera seeds. In this, the preliminary investigations into the possible use of Moringa oleifera seed suspension for the softening of hard water are presented. Four water sources: synthetic water (distilled water spiked with calcium chloride), naturally hard surface water and groundwater from two tube wells at different locations were used for the study. Modified laboratory jar test procedures for coagulation studies were used for the experimental runs. Water hardness from the sources varied from 300 up to 1000 mg/l as CaCO3. The mechanism for softening was found to be due to adsorption with the adsorption isotherm approximating to the Langmuir type, and conversion of soluble hardness-causing ions to insoluble products by precipitation reactions.
Ibrahim S. Al-Mutaz et al (2004)13., studied that the Silica removal during Lime Softening in Water Treatment Plant. In this Silica, SiO2, is typically found in well water supplies. Most of the silica found in well waters is result of dissolving silica-containing rock. Silica content in brackish water is generally in the range of 20 to 60 ppm. In Salbukh water treatment plant, silica concentration of raw water is about 30 ppm. High concentration of silica causes membrane fouling in the reverse osmosis units. During lime softening process calcium and magnesium are precipitated. Calcium is deposited as calcium carbonate while magnesium is deposited as magnesium hydroxide. Upon precipitation, magnesium hydroxide forms larger floc that more readily adsorbs or entraps silica particles in the water.
Sutarno et al (2004)25., posed in his project that Faujasite was hydrothermally synthesized from fly ash at 1000C in alkaline solution by reflux with various concentration of HCl and fusion with NaOH (weight ratio of NaOH/fly ash = 1.2) pretreatments.Thermal stability of faujasite from fly ash was tested at 400-900oC and was compared with commercial zeolite Y. The solid products were characterized by X-ray diffraction method, chemical analysis and nitrogen adsorption (BET). Results showed that synthesis of faujasite from fly ash via fusion pretreatment with NaOH has resulted faujasite selectively, however, the faujasite obtained still showed relatively low thermal stability due to its relatively low of Si/Al mol ratio.
Doungmanee Rungsuk et al (2006)6., goes with the Studies on Coal fly ash (CFA) was used to synthesize zeolite by fusion method. The synthesis conditions were optimized to obtain the product with high cation exchange capacity (CEC). The fusion temperature that resulted in the best CEC result was 450oC. The optimal fusion time was found to be 2 hours and this gave the highest CEC of 219.7 meq/100g. The zeolite product was characterized using various techniques, i.e. X-ray diffraction scanning electron microscopy, and the results revealed that the zeolite of this work was closest to the property of the X- type zeolite.
Tembhurkar. A.R et al (2006)26., goes with the Studies on Fluoride Removal Using Adsorption process. Batch adsorption studies were undertaken to assess the suitability of commercially available activated charcoal to remediate fluoride-contaminated water. The effects of some of the major parameters of adsorption, viz. pH, and dose of adsorbent, rate of stirring, contact time and initial adsorbate concentration on fluoride removal efficiency were studied and optimized. The optimum sorbent dose was found to be 2.0 g/100 mL, equilibrium was achieved in 120minutes and enhanced adsorption was obtained at pH 2. Maximum fluoride removal was observed to be 94% at optimum conditions. Freundlich as well as Langmuir isotherms were plotted and kinetic constants were determined.
Faizul Che Pa et al (2007)7., goes with the Studies on Fly ash (PFA) is a complex material produced after combustion in coal-fired power plants. About half of this fly ash is disposed as solid wastes. A possible alternative to disposal of the fly ash is the synthesis of zeolite. Zeolite Boggsite (Na37Ca74Al185Si775O192 7H2O) was synthesized from fly ash by hydrothermal treatment with NaOH solutions as identified by x-ray diffraction. The zeolite type and degree of crystallization were found to be dependent on the reaction conditions and mineralogy of the raw material, particularly in terms of the relative concentrations of SiO2 and Al2O3.
Balaji. R et al (2008)4., studied the removal of Iron from drinking / ground water by using agricultural waste as natural adsorbents. The sustainability of good health depends upon the purity of water. However groundwater may be exposed towards to contamination by various anthropogenic activities such as agricultural, domestic and industrial. Groundwater quality problem are typically associated with high level of iron concentration in West Mugapair, Chennai. The normal drinking water contains permissible limit of iron concentration 0.3mg/L but the West The parameters such as effect of pH, adsorbent dosage, contact time, initial concentration, etc., were studied. Maximum removal of total iron was observed in the pH range of 4-5. The highest percentage removal of total iron was observed at Coconut coir (96%) compared than sugarcane bagasse (93%).
Hung-Der Hsu et al (2008)12., studied the Silica pre-treatment for RO membrane b softening-adsorption. It states that previous literature has proven that both softening species of CaCO3(s) and Mg(OH)2(s) are capable of removing silica, but the mechanisms were not clearly described. Three different possible removal mechanisms were discussed here: Adsorption by aged CaCO3(s) and Mg(OH)2(s), Adsorption by amorphous (freshly precipitated) CaCO3(s) and Mg(OH)2(s) and Co-precipitation of CaCO3(s) and Mg(OH)2(s). A synthetic silica water was used to distinguish the above three mechanisms and the groundwater with 21 mgL-1 silica and high hardness was also used for comparison.
Winn-Jung Huang et al (2008)27., studied the effect of characteristics of activated carbon on removal of Bromate. This study evaluates how the characteristics of activated carbon (AC) influence the adsorption–reduction of Bromate (BrO3-) by performing kinetic and isotherm tests. Experimental results reveal that both physical and chemical effects simultaneously affect the adsorption–reduction process. The wood-based carbons contained more mesopores than coconut- and coal-based carbons, resulting in the adsorption of more BrO3-. The equilibrium and maximum adsorption capacities were calculated as a function of the effect of mesopore volume.
Gagik Badalians Gholikandi et al (2010)8., studied the natural Zeolites Application as sustainable adsorbent for heavy metals removal from drinking water. There are great resources of natural Zeolite in Iran. Zeolite, an inorganic ion-exchanger, may be used as a suitable technical-economical solution for water treatment in many regions of Iran. In this study, the characterization of natural Zeolite Natrolite and the feasibility of removing hardness, cations, and anions were investigated. First, zeolite composition and type were determined using X-ray Fluorescence (XRF), thermal analysis, and infrared spectrometer. However, by pH increases in the water sample, the arsenate anion removal arbitrarily changed: less in pH=3. But the removal efficiency got better in alkaline pH. It is obvious that by increasing the pH of water samples, the removal efficiency will decrease. In spite of that, the removal efficiency of chromate ion, in acidity pH, was more than Alkaline. The maximum removal of Cr occurred in acidic media at pH<1.5
Gamal O. El-Sayed (2010)9., studied the removal of water hardness by adsorption on Peanut hull. Studies on a batch sorption process using peanut hull as a low cost sorbent was investigated to remove calcium and magnesium ions from aqueous solutions. The influence of operational conditions such as contact time, metal ion initial concentration, sorbent mass, solution initial pH, agitation speed, ionic strength and temperature, on the sorption kinetics of calcium and magnesium were studied.
Anburaj Raj et al (2011)2., studied the Calcium removal from aqueous solution by marine Cyanocobacterium, Gloeocapsa species: adsorption kinetics and equilibrium studies. Calcium is an essential macronutrient required for healthy human life. It is abundantly present in seawater and its extraction is possible through biological means by using cyanobacteria. Efficiency of the marine cyanobacteria in removal of calcium is little known. Hence, the present work analysed adsorption kinetics of calcium from aqueous solution by using the marine cyanobacterium (Gloeocapsa sp.) in batch culture methods. The effect of initial calcium concentration, temperature, and adsorbent mass on calcium removal was tested. The process of calcium adsorption followed pseudo second-order rate expression and obeyed the Langmuir’s model with high correlation coefficient (R2>1). The results indicate that Gloeocapsa species is a potential adsorbent for calcium, as evident by its high calcium adsorption value under optimal culture conditions.
Jagdish Singh et al (2011)14., studied the dynamics of adsorption isotherms for the treatment conductivity and hardness of effluent with novel isolated microorganism. Microorganisms possess a capacity to accumulate organic and inorganic matter in their body, evoke the process of their enzymatic transformation and adsorb them on the cell surface. Detailed batch studies with the selected JR11 microbial biomass as a adsorbent, has been carried out to investigate the effect of pH, contact time on the adsorption isotherm for Hardness and Conductivity. Isolated bacterial strains JR11 posses the capacity to reduce Hardness and Conductivity of water about 80±2 % at pH 7 with contact time of 85 minutes. The adsorption kinetic study satisfied the model Langmuir and Freundlich equilibrium constant. Adsorption capacity was found to be 47.2 and 26 mg/g of adsorbent for Hardness and Conductivity respectively.
Bhaumik. R et al (2012)5., studied that the Eggshell powder as an adsorbent for removal of Fluoride from aqueous Solution: equilibrium, kinetic and thermodynamic Studies. He states that a new medium, eggshell powder has been developed for fluoride removal from aqueous solution. Fluoride adsorption was studied in a batch system where adsorption was found to be pH dependent with maximum removal efficiency at 6.0. The experimental data was more satisfactorily fitted with Langmuir isotherm model. The kinetics and the factor controlling adsorption process fully accepted by pseudo-second-order model were also discussed. Ea was found to be 45.98 kJmol-1 by using Arrhenius equation, indicating chemisorption nature of fluoride onto eggshell powder.
Pankaj Garg et al (2012)19., studied the Adsorption of Fluoride from drinking water on Magnesium substituted Hydroxyapatite. In the present study performance of synthesized adsorbent was investigated for fluoride removal from drinking water under batch mode operations. Magnesium substituted Hydroxyapatite (Mg-HAp) which is a calcium-magnesium based adsorbent was characterized using XRD. The effect of different parameters such as pH, initial fluoride concentration, contact time and co-existing ions (Bicarbonate, Sulphate, Chloride, Nitrate) were studied to understand the adsorption behaviour of sorbent under various conditions. Adsorption kinetics followed a typical kinetic curve and pseudo second order rate model described the kinetic sorption process indicating that chemical adsorption, rather than mass transfer, is the rate determining step. Equilibrium was reached in less than 24 hours of contact time and equilibrium data followed Freundlich isotherm model more closely as compared to Langmuir isotherm model.
Ahmed Mahdi Saeed et al (2013)1., studied the new approach for removal of total hardness (Ca2+, Mg2+) from water using commercial polyacrylic acid hydro gel beads, study and application. Adsorption and water treatment of Ca (II) and Mg (II) hardness were investigated via adsorption of metal ions onto commercial polyacrylic acid hydro gel beads as a novel sorbent for metal ions (Ca2+, Mg2+) removal and water treatment. Batch equilibrium technique was carried out under the influence of solution pH, contact time, sorbent dosage, initial metal concentration and competitive study. The maximum metal ions capacity (Ca2+, Mg2+) values were identified as 171.2 and 193.6, respectively. Sorption equilibrium was established in 24 hr and the data were described by both Langmuir and Freundlich models. The potential application of this sorbent for water treatment and metal ions removal from water samples is successfully accomplished.
Ashraful Islam et al (2013)3., studied the removal of pH, TDS (total dissolved solids) and Colour from Textile Effluent by Using Coagulants and Aquatic/Non Aquatic Plants as Adsorbents. The pH of Textile effluent is generally high because of use of many alkaline substances in Textile processing. The total dissolved solids (TDS) are those solids remain as soluble form in Textile effluent. There are several methods available for removal of TDS and colour from Textile effluent such as, ion exchange, coagulation and flocculation, biological decolourization, adsorption etc. Among all these methods adsorption is still a procedure of choice for TDS and colour removal. Several naturally occurring aquatic/non aquatic plants have been used in this work as adsorbents. These were water hyacinth, water lily and bark of plantain plant (banana).
Gandhi. N et al (2013)11., studied the adsorption studies of Chromium by using low cost adsorbents. The presence of toxic heavy metals such as Chromium contaminants in aqueous streams, arising from the discharge of untreated metal containing effluents into water bodies, is one of the most important environmental problems. Adsorption is one of the effective techniques for Chromium removal from wastewater. In the present study, adsorbent was prepared from low cost adsorbents and studies were carried out for Chromium removal. Batch adsorption studies demonstrated that the adsorbents had significant capacity to adsorb the Chromium from aqueous solution. It was found that the adsorption increased with increase in contact time and adsorbent dosage. An attempt was made to study mixed Algae as an adsorbent for removal of Chromium.
Mohammad Noori Sepehr (2013)17 et al., studied the removal of hardness agents, Calcium and Magnesium, by natural and Alkaline modified Pumice stones in single and binary systems. Natural and alkaline modified pumice stones were used for the adsorption of water hardening cations, Ca+2 and Mg+2. The adsorbents were characterized using XRF, XRD, SEM and FTIR instrumental techniques. At equilibrium time and for 150 mg/L of a given cation, removal efficiencies were 83% and 94% for Calcium and 48% and 73% for Magnesium for raw and modified Pumices, respectively. The optimal pH for raw and modified Pumices were found to be 6.0, leading to the removal of 79 and 96% of Calcium and 51 and 93% of Magnesium by 10 g/L of raw and modified Pumice adsorbents, respectively.
Patil. I.D et al (2013)20., studied the ground water nitrate removal by using ‘Chitosan’ as an adsorbent. Environmental pollution is the most terrible ecological crisis to which we are subjected today. Today the environment has become foul, contaminated, undesirable, and therefore harmful for the health of living organisms, including man. The most common contaminant identified in ground water is dissolved nitrogen in the form of Nitrate (NO3).Decomposition of organic matter present in soils leaching, of soluble fertilizers, human and animal excreta are the source of nitrate in subsurface waters. This research work is for to find preventive measures to avoid the nitrate pollution. This paper explains suitability of naturally available adsorbent i.e. ‘Chitosan’ for removal of nitrates from water is studied. The work is for the use of Chitosan in the different forms and concentrations to achieve the task.
Ravi Kumar. K et al (2013)21., studied the heavy metal removal from water using Moringa oleifera Seed coagulant and double filtration. The quality and accessibility of drinking water are of paramount importance to human health. Drinking water may contain disease-causing agents and toxic chemicals and to control the risks to public health, systematic water quality monitoring and surveillance are required. Thousands of chemicals have been identified in drinking water supplies around the world and are considered potentially hazardous to human health at relatively high concentrations. Heavy metals are the most harmful of the chemical pollutants and are of particular concern due to their toxicities to humans. Moringa oleifera seed acts as a natural coagulant, adsorbent and antimicrobial agent. It is believed that the seed is an organic natural polymer.
Satyanarayana. P et al (2013)22. studied the urban groundwater quality Assessment: A Case Study of Greater Visakhapatnam Municipal Corporation Area (GVMC), Andhra Pradesh, India. Water is indispensable for the existence and survival of life on earth. Water is needed in almost every sphere of human activity. Groundwater pollution is one of the environmental problems in urban areas. The paper presents urban groundwater quality assessment and analyzed for their physicochemical characteristics. The results of this analysis were compared with the water quality standards of WHO, BIS and CPHEEO. The water samples have been collected from 21 bore wells in GVMC area in Visakhapatnam city.
Kannan. D et al (2014)15., studied the removal of hardness (Ca2+, Mg2+) and Alkalinity from ground water by low cost adsorbent using Phyllanthus emblica wood. The main problem of the locality is the drinking water certain health problems are associated with people living in hills that are because of the presence of excess of ions and other impurities. The present study was conducted to analyze the some physicochemical parameters and removal of ions using Phyllanthus emblica adsorbent for purification of ground water for drinking purpose. In present study various dose of Phyllanthus emblica carbon are taken and checked for the efficiency dose on ground water. After treatment of water sample with Phyllanthus emblica carbon were analyzed for different parameters like TDS, Mg2+, Ca2+, Alkalinity, electrical conductivity all parameters were reduced with increased dose of Phyllanthus emblica carbon.
Khadse Shaila et al (2014)16., studied that the Synthesis of Zeolite using Fly ash and its application in Removal of Cu2+, Ni2+, Mn2+ from Paper Industry Effluent. In this paper An improved synthesis for fly ash based Zeolite has been attempted and studies have been carried out for the removal of Cu2+, Ni2+ and Mn2+ from paper industry wastewater. The parameters affecting adsorption process, such as initial pH, weight of Zeolite, contact time were investigated. The transition metal ions present in the waste Cu2+, Ni2+ and Mn2+ shows better adsorption capacities on synthesized zeolite. The order of removal of heavy metal ions is Ni2+ > Mn2+ > Cu2+.
Mohammad Almjadleh et al (2014)18., studied the use of natural and modified Jordanian Zeolitic tuff for removal of Cadmium from aqueous solutions. The possibility of using natural and modified Jordanian Zeolite for removal of Cadmium as a model component of heavy metals has been considered. Bench-scale tests using both aqueous solutions and real water under different experimental conditions have been employed. Different parameters, including metal ion concentration, modifier type, water quality and pH, have been studied. Synthetic Zeolite Molecular Sieve (4A) as control material has been also considered for the purpose of comparison. Hardness in water was found to highly impede adsorption. Sorption equilibrium was achieved within 250 min. The equilibrium isotherm data of Cadmium Zeolitic tuff were represented by Langmuir and Freundlich isotherm models, but they were well represented by Langmuir model.
Shailey Singhal et al (2014)23., studied the Bio-adsorbent: A cost-effective method for effluent treatment. Contamination of water with unwanted particles is a challenging problem for maintaining the quality and hygiene of water. Banana peels, the fruit waste were used to prepare environment benign bio-adsorbent for the adsorption of impurities from aqueous solution. They were washed, cut, dried and ground into powder of sieve size 150-212 μm before being used in the treatment of industrial water. Important parameters such as pH, colour, odour, alkalinity, hardness, residual free Chlorine, turbidity, total dissolved solids, chloride, fluoride, sulphate, metal ions, dissolved oxygen, biological Oxygen demand, chemical Oxygen demand and heavy metals were evaluated before and after the processing of water through bio adsorbent. Besides obtaining positive results in all parameters, bio adsorbent was found to be effective especially in the removal of iron and arsenic under specified conditions reaching a value of 30.45% and 25.12% respectively. The process provides a very low cost technique for one of the most challenging problem of world.
Gandhi. N et al (2014)10., studied the adsorption of Chromium from aqueous solution by using Multani mitti. The urbanization rate in India is very fast. It has increased from 10.84 to 28.5% up to 2001. Unregulated growth of urban area cause many problems. Due to increase in population, urbanization and industrialization many waste materials are entering into waste water streams and water streams causing deadful diseases. One of the most toxic chemical which is entering into the surface water is hexavalent ChromiumThe study is carried out with respect to contact time, concentration, dosage, effect of pH and temperature. The experimental data tested with adsorption isotherm and kinetics studies.
Among all of those researches mentioned above, some of them are expensive in adsorbents and processes and no one in them are research about the effectiveness of one adsorbent over other adsorbents which are obtained from different sources. So, it is cleared that we have to find a most cost effective adsorbent which is highly effects the removal of hardness from water.
3. METHODOLOGY
3.1. Methods:
The method used in this study is Adsorption method which is an hardness removal and less time taking method. The removal efficiencies are found out by using EDTA method.
3.2. Materials:
Material which is used majorly in the preparation of Laboratory Prepared Zeolite is Fly Ash and the chemicals used are Sodium Carbonate, Sodium Hydroxide, Sodium Silicate, Sodium Alignate and Lime.
For the efficiency comparison with the Laboratory prepared Zeolite, three commercial adsorbents are used in the study. They are Commercial Zeolite, Activated Carbon and Silica gel.
The major ingredient Fly ash which is used in the present adsorbent preparation is briefly described below:
Fly Ash:
Fly Ash is one of the residues generated in combustion and comprises the fine particles that rise with the flue gases. Ash which does not rise is termed bottom Ash. In an industrial context, Fly Ash is usually refers to ash produced during combustion of coal. Fly Ash is generally captured by Electrostatic precipitators or other particle filtration equipment’s before the flue gases reach the chimneys of coal-fired power plants, and together with bottom Ash removed from the bottom of the furnace is in the case jointly known as coal Ash. Depending upon the source and makeup of the coal being burned, the components of the Fly Ash vary considerably, but all Fly Ash includes substantial amounts of Silicon dioxide (SiO2), Al2O3 and Calcium Oxide(CaO), both being endemic ingredients in many coal-bearing rock strata.
Fly Ash particles are either solid or hollow and mostly glassy in nature. The particle size distribution of most bituminous coal Fly Ashes is generally similar to that of silt. Rapid cooling off from the molten state as it leaves the flame causes Fly Ash to be predominately non crystalline with minor amounts of crystalline constituents, such as Mullite, Quartz, Magnetite and Hematite.
Other constituents which may be present in high Calcium Fly Ash include, Anhydrite, Lime, Alkali Sulphate, Melilite, Nephiline, Sodalite, C3S and C2A. Fly Ash as a material is siliceous are Aluminous with pozolonic properties. It is refractory and Alkaline in nature. The shape, fineness, particle size distribution, density and composition of Fly Ash particles influence the properties of end use products. Fly Ash produced at different power plants or at the one plant with different coal sources may have different colors. Although there may be difference in the Fly Ash from one plant to another, day to day variations in the Fly Ash from a given power plant are usually quite predictable, provided plant operation and coal source remain constant. However, there can be a sustainable variation in Fly Ash obtained from burning coal with other fuels or with other combustible materials. As long as the basic operating parameter at the power plant does not change, Fly Ash from a known source supplied by a reputable Ash marketing organization should be a consistent, qualify controlled product.
The ways of Fly Ash utilization include (approximately in order of decreasing importance):
Ø Concrete production, as a substitute material for Portland cement and sand.
Ø Embankments and other structural fills(usually for road construction).
Ø Waste stabilization and solidification.
Ø Cement clinkers production-(as a substitute material for clay).
Ø Mine reclamation.
Ø Stabilization of soft soils.
Ø Road sub base construction.
Ø As aggregate substitute material (e.g. for brick production).
Ø Mineral filler in Asphaltic concrete.
Ø Agriculture uses soil amendment, fertilizer, cattle, feeders, soil stabilization in stock feed yards, agricultural stakes.
Ø Loose application on roads and parking lots for ice control.
More recently, Fly Ash has been used as a component in Zeolites.
All Chemicals and materials required for the EDTA test and adsorbtion method were taken from Vijayawada, Andhra Pradesh, India respectively. The materials are in powdered form with the fine size and used directly without any further grinding and sieving.
3.3. Adsorbents:
Synthetic hard water has prepared as instructed by Window on State Govt, where by 1g of cacl2 dissolved in a liter of deionized water to make water with hardness of 1000 mg/L as CaCl2 equivalent and this served as a stock solution. The initial concentrations are thus changed by varying the mix dosage of CaCl2.
3.4. Preparation of the Adsorbent LPZ:
Fly Ash contains Silica, Alumina with other impurities in small quantities. Analysis of Fly Ash sample used in the present study is shown in table 3.1 and the composition of Indian Fly Ash is shown in table 3.2.Silica and Alumina contained generally in the form of mixed Calcium and Aluminium Silicates.
Table 3.1 Chemical Composition of Fly Ash used in the present study
Table 3.2 Chemical Composition of Indian Fly Ash
Fly Ash contained from nearby power plant (Vijayawada) is washed thoroughly and dried to obtain constant weight. Thus prepared sample is used in preparation of Zeolite by high temperature heat treatment.
Fly Ash and Sodium Hydro oxide (NaoH) is taken in ratios of , 10:90, 15:85, 20:80, 30:70 and kept in the furnace at different temperatures 300, 400, 500, 600, 700oC for heat treatment for 2hrs. Ions of Na+ replaced surface ions of Ca2+/Mg2+ at these temperatures.
Once the activation time was reached, the contents were cooled. The Zeolite material was then washed with distilled water until residual NaoH was fully removed. Thus the sample is filtered & dried in an oven at 100oC for 2hrs and its CEC is determined to check the Zeolite content.
The treated Fly Ash is made into pellets by agglomeration with Lime, Sodium Silicate and Sodium Alignate. Now the pellets are taken in bed and same procedure is carried out by running hard water through a column and collected water is analyzed for Zeolite CEC.
The Zeolitic sample tested for cation exchange capacity by the following method.
3.5. Testing of LPZ for CEC:
1g of Zeolite is taken in a Conical Flask and 20 mL of raw water is added and shaken thoroughly for 5 minutes in orbital shaker. The treated Zeolite is filtered and washed several times with DM water. The filtrate and washing are made into standard volume and estimated for total Ca2+, Mg2+ ions by EDTA method as obtained and noted as V1. Following the same procedure,25 mL of raw water is estimated for its Ca2+,Mg2+ ions content and noted as V2.
Difference of the above values represented m mole of Ca2+/kg of Zeolite. It is observed that raw Fly Ash contains 130 m mole kg-1 while for the present Zeolite ranges from 1560-7500 m mole kg-1 depending on the treatment it has undergone.
As Fly Ash contains mixed Silicates of Sodium and Aluminium but the exact values depend on the coal from Fly Ash have been generated and temperatures through which it has been passed. In the present study, Fly Ash and NaoH is taken in different temperatures 300, 400, 500, 600, 700oC. Part of NaoH attached to Fly Ash enhancing Si:Na ratio. Thus Fly Ash is expected to be of Sodium Alumino Silicate with replaceable charges. Exact structure elucidation is complex procedure but can be observed by measuring cation exchange capacity. Again the procedure is carried out until regenerated sample, CEC is determined, which is done in present study by the removal of hardness to water.
3.6. Determination of total hardness
Principle:
A water sample is buffered to pH 10 and taken to into a Conical Flask. If an indicator dye like EBT, when added to a solution containing Calcium and Magnesium ions, the colour the solution turns to wine red.
EDTA, the titrant, complexes the Magnesium and Calcium ions removing them from association with the indicator.
When all the ions of Ca and Mg are complexed with EDTA, the indicator will turn blue. This is the end point of the titration.
Apparatus required:
Ø Burette with Burette stand,
Ø Pipettes with elongated tips,
Ø Conical Flask(Erlenmeyer Flask),
Ø 250 mL graduated cylinders,
Ø Standard Flask,
Ø Wash bottle,
Ø Beaker.
Normal fiter paper
Chemicals required:
Ø Ammonium Chloride,
Ø Ammonium Hydroxide,
Ø EDTA(Disodium salt of EDTA),
Ø Erichrome Black T,
Ø Magnesium Sulphate.
Testing of water sample:
Ø Rinse and fill the burette with 0.02M EDTA solution and adjust it to zero, then fix it to Burette stand.
Ø Take out 20 mL of hard water sample into pipette and transfer it to a clean 250 mL Conical Flask.
Ø Add 2mL of Ammonia buffer solution to the water sample so that the pH will be maintained between 9 and 10.
Ø Add few drops of EBT indicator to the Conical Flask and the sample turns to wine red in colour.
Ø Titrate the sample against the EDTA solution in the Burette till all Calcium and Magnesium ions present the sample reacts with the EDTA. The appearance of blue colour indicates that Ca and Mg ions are complexed with EDTA and forms a metal EDTA complex that is the end point of the titration.
Ø Note down the burette reading.
Ø The value of the titration is noted in mL
Ø Repeat the titration for concordant value.
Finding the total hardness by using the following formula (Eq.1):
Total hardness = (V1*N*50*1000)/(V2) mg/L (1)
Here V1 is the volume of EDTA (mL),
V2 is the volume of sample taken (mL),
N is the normality of EDTA = 0.02N,
50 is the equivalent weight of CaCL2 (mg).
3.7. Batch experiments:
All experiments were conducted in batch mode in 250 mL conical flasks. Several operating parameters including adsorbent mass (1-15 g/L), initial ion concentrations (100-1500 mg/L) and contact time (1-15 minutes) were investigated. Optimized adsorption times for Commercial Zeolite, LPZ, Activated Carbon and Silica gel adsorbents were first examined by varying the contact time at room temperature for an adsorbent mass of 1g/L of solution. For this purpose, 1g of adsorbent were added to 1L of solution in a conical flask containing Ca+2 or Mg+2 cations at a concentration in the range of 1500 mg/L. The mixture was then shaken at 480 rpm. Samples were taken at predetermined time intervals, filtered by normal filter papers in order to reduce the filtration losses and the ion concentration was measured by a titration method.
After finding the optimum time and the optimum dosage, fix the optimum time, dosage values and find the removal efficiencies at various initial ion concentrations like 100, 200, 400, 700, 1000, 1200, 1500 mg/L respectively by the four adsorbents. The removal efficiency (RE) was determined as follows (Eq. 2):
Removal efficiency (%) = (Ci-Ce) / Ci*100 (2)
The quantity of adsorbed hardness on the adsorbate; i.e. uptake was calculated by the difference of initial and final (or) equilibrium concentrations following the equation (Eq. 3):
qe = (Ci-Ce)*V/m (3)
In the above equations:
Ci is the initial ion concentration (mg/L),
Ce is the equilibrium (or) final ion concentration (mg/L),
qe is the quantity of hardness adsorbed on the adsorbate (mg/g),
m is the amount of adsorbate (g),
V is the volume of the solution (L)
Several batch experiments were conducted to find the optimum time for the four adsorbents. By using the optimum time, we find the optimum dosage required by the four adsorbents, finally, we find the removal efficiency for various initial ion concentrations by the four adsorbents.
4. RESULTS AND DISCUSSIONS
The effects of three different factors on the adsorption were investigated. These factors included the amounts of adsorbent, contact time, initial concentration of water sample. The results evaluated are based on EDTA method.
The removal efficiency values are listed in the table, which are followed on the numbering. The relevant figure, which shows the graphical representation of the effect contact time, adsorbent dose, initial concentration on the hardness removal are also listed number wise.
The adsorption of hardness ions on to the adsorbent prepared in the laboratory and gives an efficiency comparison with commercially available adsorbents.
4.1. Effect of contact time on hardness removal:
A). Commercial Zeolite:
a) Data:
Initially the water sample has a concentration of 1500 mg/L, by using Commercial Zeolite at an initial dosage of 1 g/L, 2 % efficiency is obtained initially by the solution with 1min shaking by using orbital shaker. After 4 min shaking, I got 10.6% efficiency which is sufficient for the regular water treatment for residential, commercial or industrial use. But, to get the equilibrium state the adsorbent requires 5 min and efficiency observed is 11.6 %. The trial is continued up to 7 min to show that the adsorbent gets an equilibrium state, the removal efficiencies at various time intervals are shown in the following table 4.1, the relevant graph is drawn and shown in fig 4.1:
Table 4.1 Data for the effect of contact time on hardness removal by Commercial Zeolite at the initial ion concentration (Ci) is 1500 mg/L:
b). Effect of contact time on hardness removal by Commercial Zeolite:
The effect of the contact time was studied at 1, 2, 3, 4, 5, 6, 7 minutes respectively. Fig 4.1 shows the relationship between contact time and hardness removal efficiency. Observation reveals that the removal of hardness ions improved by increasing the contact time. The percentage hardness removal approached equilibrium within 5 minutes. Further increase in contact time does not show significant change in hardness. This might be due to fact that, large number of vacant surface sites is available for the adsorption during the initial stage and with the passage of time. After some times, repulsive forces between solute molecules on solid phase and liquid phase create difficultness for the solute molecules to occupy remaining vacant surface sites.
Fig 4.1 Effect of contact time on hardness removal by Commercial Zeolite: Hardness 1500 mg/L, Dose=1g/L.
B). Laboratory prepared Zeolite:
a) Data:
Initially the water sample has a concentration of 1500 mg/L, by using LPZ at an initial dosage of 1 g/L, 2 % efficiency is obtained initially by the solution with 1min shaking by using orbital shaker. After 5 min shaking, I got 13 % efficiency which is sufficient for the regular water treatment for residential, commercial or industrial use. But, to get the equilibrium state the adsorbent requires 6 min and efficiency observed is 17 %. The trial is continued up to 8 min to show that the adsorbent gets an equilibrium state, the removal efficiencies at various time intervals are shown in the following table 4.2, the relevant graph is drawn and is shown in fig 4.2:
Table 4.2 Data for the effect of contact time on hardness removal by LPZ:
b). Effect of contact time on hardness removal by Commercial LPZ:
The effect of the contact time was studied at 1, 2, 3, 4, 5, 6, 7, 8 minutes respectively. Fig 4.2 shows the relationship between contact time and hardness removal efficiency. Observation reveals that the removal of hardness ions improved by increasing the contact time. The percentage hardness removal approached equilibrium within 6 minutes. Further increase in contact time does not show significant change in hardness. This might be due to fact that, large number of vacant surface sites is available for the adsorption during the initial stage and with the passage of time. After some times, repulsive forces between solute molecules on solid phase and liquid phase create difficultness for the solute molecules to occupy remaining vacant surface sites.
Fig 4.2 Effect of contact time on hardness removal by Commercial LPZ: Hardness 1500 mg/L, Dose=1g/L.
C) Activated Carbon:
a) Data:
Initially the water sample has a concentration of 1500 mg/L, by using Activated Carbon at an initial dosage of 1 g/L, 4 % efficiency is obtained initially by the solution with 1min shaking by using orbital shaker. After 8 min shaking, I got 15.66% efficiency which is sufficient for the regular water treatment for residential, commercial or industrial use. Also, to get the equilibrium state the adsorbent requires 9 min and efficiency observed is 16.33%. The trial is continued up to 11 min to show that the adsorbent gets an equilibrium state, the removal efficiencies at various time intervals are shown in the following table 4.3, the relevant graph is drawn and is shown in fig 4.3:
Table 4.3 Data for the effect of contact time on hardness removal by Activated Carbon:
b). Effect of contact time on hardness removal by Activated Carbon:
The effect of the contact time was studied at 1, 2, 3, 4, 5, 6, 7, 8, 9, 10, 11 minutes respectively. Fig 4.3 shows the relationship between contact time and hardness removal efficiency. Observation reveals that the removal of hardness ions improved by increasing the contact time. The percentage hardness removal approached equilibrium within 9 minutes. Further increase in contact time does not show significant change in hardness. This might be due to fact that, large number of vacant surface sites is available for the adsorption during the initial stage and with the passage of time. After some times, repulsive forces between solute molecules on solid phase and liquid phase create difficultness for the solute molecules to occupy remaining vacant surface sites.
Fig 4.3 Effect of contact time on hardness removal by Activated Carbon: Hardness 1500 mg/L, Dose=1g/L.
D). Silica gel:
a) Data:
Initially the water sample has a concentration of 1500 mg/L, by using Commercial Zeolite at an initial dosage of 1 g/L, 6% efficiency is obtained initially by the solution with 0.25 min shaking by using orbital shaker. After shaking0.5 min, I got 8% efficiency which is sufficient for the regular water treatment for residential, commercial or industrial use. But, to get the equilibrium state the adsorbent requires 0.5 min and efficiency observed is 8 %. The trial is continued up to 3 min to show that the adsorbent gets an equilibrium state, the removal efficiencies at various time intervals are shown in the following table 4.4,the relevant graph is drawn and shown in fig 4.4:
Table 4.4 Data for the effect of contact time on hardness removal by Silica gel:
b). Effect of contact time on hardness removal by Silica gel:
The effect of the contact time was studied at 0.25, 0.5, 1, 2, 3 minutes respectively. Fig 4.4 shows the relationship between contact time and hardness removal efficiency. Observation reveals that the removal of hardness ions improved by increasing the contact time. The percentage hardness removal approached equilibrium within 0.5 minutes. Further increase in contact time does not show significant change in hardness. This might be due to fact that, large number of vacant surface sites is available for the adsorption during the initial stage and with the passage of time. After some times, repulsive forces between solute molecules on solid phase and liquid phase create difficultness for the solute molecules to occupy remaining vacant surface sites.
Fig 4.4 Effect of contact time on hardness removal by Silica gel: Hardness 1500 mg/L, Dose=1g/L.
4.2.Effect of Adsorbent dose on hardness removal:
A). Commercial Zeolite:
a) Data:
Initially the water sample has a concentration of 1500 mg/L, by using Commercial Zeolite at contact time of 5 min, 11.66% efficiency is obtained initially by the solution with the dosage of 1g/L. To get the equilibrium state the adsorbent requires 4 g/L and efficiency observed is 14.66 %. The trial is continued up to a dosage of 8 g/L to show that the adsorbent gets an equilibrium state, the removal efficiencies at various adsorbent dosages are shown in the following table 4.5, and the relevant graph is drawn and shown in fig 4.5:
Table 4.5 Data for the effect of adsorbent dose on hardness removal by Commercial Zeolite:
b). Effect of adsorbent dose on hardness removal by Commercial Zeolite:
The effect of the adsorbent dose on hardness removal is shown in Fig 4.5. Adsorbent dose was varied between 1-8 g/L and it was indicated that the removal efficiency of hardness improved by increasing the adsorbent dose up to 4 g/L where further dose increase yields negligible adsorption. Greater availability of exchangeable sites at higher concentration of adsorbent is the reason for the increase in hardness removal with the increase in adsorbent dose. According to Chakrabarthy and Sharma, after a certain dose of adsorbent, the maximum adsorption is attained and hence the amount of ions remains constant even with further addition of adsorbent. That’s why beyond 4 g/L, the adsorption found to be constant.
Fig 4.5 Effect of adsorbent dose on hardness removal by Commercial Zeolite: Hardness 1500 mg/L, Contact time=5minutes.
B). Laboratory Prepared Zeolite:
a) Data:
Initially the water sample has a concentration of 1500 mg/L, by using Laboratory prepared Zeolite at contact time of 6 min, 17% efficiency is obtained initially by the solution with the dosage of 1g/L. To get the equilibrium state the adsorbent requires 5 g/L and efficiency observed is 30%. The trial is continued up to a dosage of 7g/L to show that the adsorbent gets an equilibrium state, the removal efficiencies at various adsorbent dosages are shown in the following table 4.6, and the relevant graph is drawn and shown in fig 4.6:
Table 4.6 Data for the effect of adsorbent dose on hardness removal by LPZ:
b). Effect of adsorbent dose on hardness removal by LPZ:
The effect of the adsorbent dose on hardness removal is shown in Fig 4.6. Adsorbent 1-7 g/L and it was indicated that the removal efficiency of hardness improved by increasing the adsorbent dose up to 7 g/L where further dose increase yields negligible adsorption. Greater availability of exchangeable sites at higher concentration of adsorbent is the reason for the increase in hardness removal with the increase in adsorbent dose. According to Chakrabarthy and Sharma, after a certain dose of adsorbent, the maximum adsorption is attained and hence the amount of ions remains constant even with further addition of adsorbent. That’s why beyond 12 g/L, the adsorption found to be constant.
Fig 4.6 Effect of adsorbent dose on hardness removal by LPZ: Hardness 1500 mg/L, Contact time=6 minutes.
C). Activated Carbon:
a) Data:
Initially the water sample has a concentration of 1500 mg/L, by using Activated Carbon at contact time of 9 min, 16.33 % efficiency is obtained initially by the solution with the dosage of 1g/L. To get the equilibrium state the adsorbent requires 2 g/L and efficiency observed is 18.66 %. The trial is continued up to a dosage of 6 g/L to show that the adsorbent gets an equilibrium state, the removal efficiencies at various adsorbent dosages are shown in the following table 4.7, and the relevant graph is drawn and shown in fig 4.7:
Table 4.7 Data for the effect of adsorbent dose on hardness removal by Activated Carbon:
b). Effect of adsorbent dose on hardness removal by Activated Carbon:
The effect of the adsorbent dose on hardness removal is shown in Fig 4.7 Adsorbent dose was varied between 2-10 g/L and it was indicated that the removal efficiency of hardness improved by increasing the adsorbent dose up to 4 g/L where further dose increase yields negligible adsorption. Greater availability of exchangeable sites at higher concentration of adsorbent is the reason for the increase in hardness removal with the increase in adsorbent dose. According to Chakrabarthy and Sharma, after a certain dose of adsorbent, the maximum adsorption is attained and hence the amount of ions remains constant even with further addition of adsorbent. That’s why beyond 4 g/L, the adsorption found to be constant.
Fig 4.7 Effect of adsorbent dose on hardness removal by Activated Carbon: Hardness 1500 mg/L, Contact time=9 minutes.
D). Silica gel:
a) Data:
Initially the water sample has a concentration of 1500 mg/L, by using Silica gel at contact time of 0.5 min, 8% efficiency is obtained initially by the solution with the dosage of 1g/L. To get the equilibrium state the adsorbent requires 4 g/L and efficiency observed is 12%. The trial is continued up to a dosage of 8 g/L to show that the adsorbent gets an equilibrium state, the removal efficiencies at various adsorbent dosages are shown in the following table 4.8, and the relevant graph is drawn and shown in fig 4.8:
Table 4.8 Data for the effect of adsorbent dose on hardness removal by Silica gel:
b). Effect of adsorbent dose on hardness removal by Silica gel:
The effect of the adsorbent dose on hardness removal is shown in Fig 4.8 Adsorbent dose was varied between 1-8 g/L and it was indicated that the removal efficiency of hardness improved by increasing the adsorbent dose up to 4 g/L where further dose increase yields negligible adsorption. Greater availability of exchangeable sites at higher concentration of adsorbent is the reason for the increase in hardness removal with the increase in adsorbent dose. According to Chakrabarthy and Sharma, after a certain dose of adsorbent, the maximum adsorption is attained and hence the amount of ions remains constant even with further addition of adsorbent. That’s why beyond 4 g/L, the adsorption found to be constant.
Fig 4.8 Effect of adsorbent dose on hardness removal by Silica gel: Hardness 1500 mg/L, Contact time=0.5 minutes.
4.3. Effect of Initial ion concentration on hardness removal:
A). Commercial Zeolite:
a) Data:
By using Commercial Zeolite at a dosage of 4g/L with an optimum contact time of 5 min, the removal efficiencies are decreased with increasing the initial ion concentrations. The percentages of removal efficiencies at various initial ion concentrations are shown in the following table 4.9,the relevant graph is drawn and shown in fig 4.9
Table 4.9 Data for the effect of initial ion concentration on hardness removal by Commercial Zeolite:
b). Effect of initial ion concentration on hardness removal by Commercial Zeolite::
The effect of the Initial ion concentration on hardness removal is shown in Fig 4.9. The initial ion concentration was varied between 100-1500 mg/L and it was indicated that the removal efficiency decreases by increasing the initial ion concentration.
Fig 4.9 Effect of initial ion concentration on hardness removal by Commercial Zeolite: Contact time=5 minutes, Dosage=4g/L.
B). Laboratory Prepared Zeolite:
a) Data:
By using Laboratory prepared Zeolite at a dosage of 5 g/L with an optimum contact time of 6 min, the removal efficiencies are decreased with increasing the initial ion concentrations. The percentages of removal efficiencies at various initial ion concentrations are shown in the following table 4.10,the relevant graph is drawn and shown in fig 4.10:
Table 4.10 Data for the effect of initial ion concentration on hardness removal by LPZ:
b). Effect of initial ion concentration on hardness removal by LPZ:
The effect of the Initial ion concentration on hardness removal is shown in Fig 4.10. The initial ion concentration was varied between 100-1500 mg/L and it was indicated that the removal efficiency decreases by increasing the initial ion concentration.
Fig 4.10 Effect of initial ion concentration on hardness removal by LPZ: Contact time=6 minutes, Dosage=5g/L.
C). Activated Carbon:
a) Data:
By using Commercial Zeolite at a dosage of 2g/L with an optimum contact time of 9 min, the removal efficiencies are decreased with increasing the initial ion concentrations. The percentages of removal efficiencies at various initial ion concentrations are shown in the following table 4.11,the relevant graph is drawn and shown in fig 4.11:
Table 4.11 Data for the effect of initial ion concentration on hardness removal by Activated Carbon:
b). Effect of initial ion concentration on hardness removal by Activated Carbon:
The effect of the Initial ion concentration on hardness removal is shown in Fig 4.11. The initial ion concentration was varied between 100-1500 mg/L and it was indicated that the removal efficiency decreases by increasing the initial ion concentration.
Fig 4.11 Effect of initial ion concentration on hardness removal by Activated Carbon: Contact time=9 minutes, Dosage=2g/L.
D). Silica gel:
a) Data:
By using Silica gel at a dosage of 4g/L with an optimum contact time of 0.5 min, the removal efficiencies are decreased with increasing the initial ion concentrations. The percentages of removal efficiencies at various initial ion concentrations are shown in the following table 4.12,the relevant graph is drawn and shown in fig 4.12:
Table 4.12 Data for the effect of initial ion concentration on hardness removal by Silica gel:
b). Effect of initial ion concentration on hardness removal by Silica gel:
The effect of the Initial ion concentration on hardness removal is shown in Fig 4.12. The initial ion concentration was varied between 100-1500 mg/L and it was indicated that the removal efficiency decreases by increasing the initial ion concentration.
Fig 4.12 Effect of initial ion concentration on hardness removal by Silica gel: Contact time=0.5 minutes, Dosage=4g/L.
4.4. Comparison of four adsorbents by their equilibrium time and dose:
The values of equilibrium times and dosages of commercial Zeolite, LPZ, Activated Carbon and Silica gel are given below; here the contact time observed from 1-10 minutes, dosages are observed from 1-15 g/L.
Table 4.13 Data for the four adsorbents by their equilibrium time and dose:
Fig 4.13 Comparison of four adsorbents by their equilibrium time and dose
4.5. Maximum percentages of removal efficiencies of four adsorbents when treated for Contact time, Dosage, Initial ion concentration.
A). Contact Time:
When the adsorbents are treated for contact time, the maximum removal efficiency percentages are listed in the table 4.14 and relevant graph is drawn and is shown in fig 4.14:
Table 4.14 Data for the maximum removal efficiencies of four adsorbents when treated for contact time:
Fig 4.14 maximum percentages of removal efficiencies of four adsorbents, when treated for Contact time.
B). Adsorbent dosage:
When the adsorbents are treated for adsorbent dosage, the maximum removal efficiency percentages are listed in the table 4.15 and relevant graph is drawn and is shown in fig 4.15:
Table 4.15 Data for the maximum removal efficiencies of four adsorbents when treated for adsorbent dosage:
Fig 4.15 maximum percentages of removal efficiencies of four adsorbents, when treated for adsorbent dosage.
C). Initial ion concentration:
When the adsorbents are treated for initial ion concentration, the maximum removal efficiency percentages are listed in the table 4.16 and relevant graph is drawn and is shown in fig 4.16:
Table 4.16 Data for the maximum removal efficiencies of four adsorbents when treated for initial ion concentration:
Fig 4.16 maximum percentages of removal efficiencies of four adsorbents when treated for Initial ion concentration.
5. CONCLUSIONS AND FUTURE SCOPE
5.1. Conclusions:
The main conclusions of the study are given below:
· The maximum hardness is removed by the Laboratory prepared Zeolite at the initial ion concentration 100mg/L .i.e, %.
· Activated carbon is highly efficient adsorbent at the higher concentration levels like 1500mg/L and the removal efficiency is 16.33%.
· The optimum adsorbent doses are 6, 12, 4 and 6 g/L, & contact times are 60, 30, 150 and 90 minutes for Commercial Zeolite, Laboratory prepared Zeolite, Activated carbon and Silica gel respectively.
· The laboratory prepared Zeolite is effective up to the concentration level of 500mg/L i.e., usable for drinking water.
5.2. Future scope:
· LPZ is effective up to 3 washes only, so, it is essential to improvise the capacity for more washes, hence to reduce the economy and for saving time.
· The continuation studies on this thesis to be concentrates on pH change, temperature variation and particle size.
APPENDIX
Sample calculation 1:
The hardness of the required sample is calculating by the following:
Determination of total hardness of the prepared stock solution:
Table 4.27 Data for finding hardness for the prepared stock solution:
Specimen calculations:
Volume of EDTA = 10.2 mL
Normality of EDTA = 0.02N
Volume of the sample =20 mL
Equivalent weight of CaCO3 = 50 mg
Total Hardness = (Volume of EDTA*N*50*1000)/Volume of the sample
Calcium hardness as CaCO3 equivalent= (10.2*0.02*50*1000)/20
= 510 mg/L as CaCO3 equivalent
The total hardness of the sample = 510 mg/L
Sample calculation 2:
Determination of adsorption capacity (qe):
The adsorption capacity of a sample with an initial ion concentration of 100 mg/L as shown below:
The required formula is
qe = (Ci-Ce)*V/m
Here, Ci = 100 mg/L
Ce = 40 mg/L
V= 1 L
M = 2g
Therefore qe = (100-40)*1/2
=60/2
=30mg/g
5. CONCLUSIONS AND FUTURE SCOPE
5.1. Conclusions:
The main conclusions of the study are given below:
· The maximum hardness is removed by the Laboratory prepared Zeolite at the initial ion concentration 100mg/L .i.e, %.
· Activated carbon is highly efficient adsorbent at the higher concentration levels like 1500mg/L and the removal efficiency is 16.33%.
· The optimum adsorbent doses are 6, 12, 4 and 6 g/L, & contact times are 60, 30, 150 and 90 minutes for Commercial Zeolite, Laboratory prepared Zeolite, Activated carbon and Silica gel respectively.
· The laboratory prepared Zeolite is effective up to the concentration level of 500mg/L i.e., usable for drinking water.
5.2. Future scope:
· LPZ is effective up to 3 washes only, so, it is essential to improvise the capacity for more washes, hence to reduce the economy and for saving time.
· The continuation studies on this thesis to be concentrates on pH change, temperature variation and particle size
In 1756, Swedish geologist Axel Cronstedt (1722–1765) best known as the pioneer of nickel—begat the name "zeolite".
Zeolites are natural volcanic minerals that are mined in specific parts of the world. At the point when volcanoes eject, liquid magma and thick cinder(ash) blasts out. Since numerous volcanoes are situated on an island or close to a sea, this magma and cinder frequently streams into the ocean.
Heat a glass of water and you'll see steam ascend off it eventually as it goes to the boil. You absolutely don't anticipate that the same thing will happen in the event that you warm a stone unless it's an exceptional sort of rock called a zeolite, which traps water inside it. since it truly signifies "bubbling stone"; today, the term alludes to more than 200 unique minerals that have a wide range of fascinating uses, from water conditioners and feline litter to creature sustenance and modern impetuses.
Zeolites structure with various crystalline structures, which have vast open pores (here and there alluded to as cavities) in an exceptionally normal game plan and generally the same size as little particles. Zeolites are hydrated aluminosilicate minerals produced using interlinked tetrahedra of alumina (AlO4) and silica (SiO4). In more straightforward words, they're solids with a generally open, three-dimensional precious stone structure worked from the components aluminum, oxygen, and silicon, with soluble base or basic Earth metals, (for example, sodium, potassium, and magnesium) in addition to water atoms caught in the holes between them.
A large portion of the zeolites found in nature are thermally steady. Some transitional stages (meta stable) can not be found in nature, in light of the fact that their security changes into the more steady one in the wake of breathing easy or augmented warming time. In research facility one can tune for the most part system sort wanted for his application, in a large portion of the cases one can likewise tune the substance creation of the structure. The metastable zeolite development can be accomplished in the lab by extinguishing the union response. Be that as it may, a portion of the zeolites are there they are combined in the research facility as of late, for example, natrolite iin 2014. Aside from this the system structure will be verging on comparable for regular and manufactured zeolite of same topology, and as a consequence of it nature of XRD example. Later can be distinctive if one modifies the substance structure (shift in top positions)... and so forth...
One more distinction is there,, in lab on can blend the huge measure of required zeolite while nature has impediments so for mechanical applications one can get mass sum from pilot plants.
Important Uses:
1.100% safe and non-dangerous at any level: All hints of the mineral are totally dispensed with out of the body inside of 6 to 8 hours. It is protected to bring with most prescriptions (the length of they are not substantial metal based), but rather as dependably you ought to check with a therapeutic expert first. *
2. Uproots substantial metals and poisons: Micronized zeolite has the ideal sub-atomic structure for catching and expelling overwhelming metals from the body without evacuating sound particles and minerals. (as clarified in chart above). Numerous individuals report feeling expanded vitality, clarity and essentialness in the wake of evacuating overwhelming metal develop. *
3. Equalizations body's pH levels to a sound alkalinity: Whenever micronized zeolite is acquainted with the body, it cradles the framework towards marginally basic (pH 7.35 to 7.45), which is an ideal pH for the human body. Zeolite draws in and after that cradles overabundance protons which cause sharpness and along these lines can help numerous conditions from heartburn to Candida and joint inflammation.
4. Zeolite is anything but difficult to take: Most detoxification items are hard to take and numerous must be infused into the blood or directed, for example, a bowel purge. Zeolite works at the cell level, has no taste or scent and it can be tackled a void stomach. (you wont even know you are taking it)
1. INTRODUCTION
1.1. Background:
Drinking water is one of the basic needs of life and essential for survival. Still more than one billion people all over the world do not have ready access to an adequate and safe water supply and more than 800 million of those unsaved live in rural areas. In India, ground water is being used as raw water for 85% public water supply. Water supply varies widely in terms of region and country. In 1970s, of the approximately 2.5 billion people in developing world, only 38% has safe drinking water. At the beginning of the 1980s, water supply coverage was 75% in urban areas and 46% in rural areas. In developing countries, 75% of the population had access to water supply. So they are always prone to loss of their lives or cost a big toll to save themselves from the occurrence of different water-borne disease.
1.2.Justification:
Water contamination due to pathogenic agents, chemicals, heavy metals, pesticides, water disinfectants, and thereby product as a consequence of industrial and agricultural activities leaching from soil, rocks, and atmospheric deposition and other human activities has become a hazard to human health in several regions of world.
Most of the water resources should be treated for purification before consumption. In some countries, groundwater is the main safe drinking water resource. In some cases, the resource does not satisfy to the desirable levels regarding their chemical properties, such as hardness, nitrate contamination, heavy metals, soluble iron, etc. Among them, water hardness can appear problematic in some cases; it can also be considered as an important aesthetic parameter.
However, because public acceptance of hardness differs remarkably according to local conditions, a maximum acceptable level has not been defined. In general, water supplies with total hardness higher than 300 mg/L can be tolerated by consumers but are considered as poor resources; while values higher than 600 mg/L are not acceptable for most of the domestic consumptions. Hardness in water refers to existing divalent ions, such as iron, manganese, calcium and magnesium. Among them, calcium and magnesium are known as the dominant species for water hardening.
Although it has been shown that water hardness doesn’t have serious health impact, it has been demonstrated that hard water is responsible for the formation of deposits in boiler and household facilities, as well as diverse influence on cleaning performances of detergents. Formation of precipitates may cause a decrease of heat transfer in boiler, a decrease of fluid rate, bursting of water pipe line, the formation of stain in dishes and clothes. Furthermore, high concentrations of Magnesium in drinking water may induce a bitter taste. In water purification and treatment plant, lime and soda ash are used for the removal of hardness. One of the main drawbacks of this process is the resulting waste, namely the large amount of liquid sludge produced, as well as the need for re-carbonation of the softened water. In addition, the use of additional chemicals to prevent sludge production is restricted and hence in most cases water hardness species cannot be fully removed. Ion exchange processes have been considered as an alternative approach which is commercially in use worldwide. However, high power consumption and periodical regeneration of saturated ion-exchanger resins are needed. Potential hazards of the effluent solutions resulting from the regeneration process should also be taken into account.
Recently, various methods including electrodeionization process, electro membrane processes, capacitive deionization, membrane and fluidized pellet reactor, ion exchange process and adsorption have been studied for the removal of a wide variety of ionic and molecular species from various water streams, including those responsible for hardness (e.g. Ca2+ and Mg2+ cations). Amongst the developed processes, adsorption has been widely studied for the uptake of various ionic and molecular species form water. Although the efficiency of activated carbon (AC) as an adsorbent, it cost remains its main drawback.
Hence, finding inexpensive and efficient adsorbents remains a topical matter to water treatment industries. Naturally occurring Zeolite have several applications in agriculture, water treatment, aquaculture, manufacturing of paper, gas adsorption and as a catalyst. Large quantities of Zeolite have been synthesized and incorporated as a part of detergents. Fly Ash has been identified for its activity similar to Zeolite and investigations were carried out further to Zeolite character of fly ash.
Zeolite have been synthesized by hydrothermal crystallization of silica gel, NaOH and Al salts. The high charge present in Zeolite is tetrahedral co-ordinates of Al which is normally takes place under high pH and temperature.
As Fly Ash contain mixed silicate of sodium and aluminium but depends on the coal from which fly ash have been generated and temperatures through which it has been passed. In the present study fly ash and alkali (Na2CO3) is taken in different ratios and thermal treatment is carried out in furnace at different temperatures 300, 400, 500, 600, 7000 C. A part of Na2CO3 attaches to fly ash enhancing Si: Na ratio. Thus formed fly ash is expected to be of sodium-alumino-silicate with replaceable charges. Exact structural elucidation is a complex procedure but Zeolite character can be quantified by measuring cation exchange capacity (CEC), which is done in present study by removal of hardness of water.
CEC values are increased with alkali and processing temperature up to certain values followed by a decrease. CEC of the values increases up to 60 minute and attain constant activity indicating maturity of product. Regeneration of each sample was carried out thrice and its values are found reduce its activity for each subsequent regeneration. However, its activity ceases to exist after three regenerations.
Zeolite is obtained out of the process is of very small particle size in the size range of less than 1 micron. Zeolite in powder form is difficult to handle, it is a major drawback in all these processes where Zeolites involved. This precludes their use in future applications such as packed bed in columns for waste water treatment. To overcome this problem, Zeolite material is subjected to size enlargement with sufficient porosity. It is made into pellets preventing it from drastic change of property.
In the present study, an attempt was made to find the efficiency of various available adsorbents in comparison with a newly prepared adsorbent in laboratory.
1.3.Objectives:
The main objectives of the study are given below: :
Ø To find the adsorptive capacity of LPZ,
Ø To find the effective time for adsorption,
Ø To determine the most cost effective adsorbent,
Ø To compare the relative merits and demerits of various adsorbents.
1.4. Limitations Of The Project:
.
All studies have limitations. However, it is important that you restrict the discussion to limitations related to the research problem under investigation. For example, if a meta-analysis of existing literature is not a stated purpose of research, it should not be discussed as a limitation. Do not apologize for not addressing issues that did not promise to investigate in Paper
Here are examples of limitations may need to describe and to discuss how they possibly impacted your findings.
Possible Methodological Limitations
The number of the units of analysis you use in your study is dictated by the type of research problem you are investigating. Note that, if sample size is too small, it will be difficult to find significant relationships from the data, as statistical tests normally require a larger sample size to ensure a representative distribution of the population and to be considered representative of groups of people to whom results will be generalized or transferred.
Lack of available and/or reliable data -- a lack of data or of reliable data will likely require to limit the scope of analysis, the size of your sample, or it can be a significant obstacle in finding a trend and a meaningful relationship. Need to not only describe these limitations but to offer reasons why you believe data is missing or is unreliable.
Lack of prior research studies on the topic -- citing prior research studies forms the basis of your literature review and helps lay a foundation for understanding the research problem you are investigating. Depending on the currency or scope of your research topic, there may be little, if any, prior research on topic. Note that this limitiation can serve as an important opportunity to describe the need for further research.
Measure used to collect the data -- sometimes it is the case that, after completing your interpretation of the findings, you discover that the way in which you gathered data inhibited your ability to conduct a thorough analysis of the results.
However, self-reported data contain several potential sources of bias that should be noted as limitations: (1) selective memory (remembering or not remembering experiences or events that occurred at some point in the past); (2) telescoping [recalling events that occurred at one time as if they occurred at another time]; (3) attribution [the act of attributing positive events and outcomes to one's own agency but attributing negative events and outcomes to external forces]; and, (4) exaggeration [the act of representing outcomes or embelishing events as more significant than is actually suggested from other data].
1.4. Organization Of Documentation:
Five chapters and an appendix make up the thesis. The introduction constitutes the first chapter and gives a background of the presence of hardness ions in the water and methods currently used their mitigation.
A summary of the relevant literature reviewed in the study is discussed in Chapter two; this chapter provides the necessary information on the success of different types of adsorbents, particularly inexpensive adsorbents used in the removal of hardness ions from water.
Chapter three provides a detailed description of the materials and methods used in the research. The types of adsorbents used to describe the adsorption of the hardness ions on to the adsorbent are described here.
Chapter four provides the results of the study as well as a comprehensive discussion of the research findings. This chapter compares and contracts the findings of this research with those of other researchers and provides explanations.
The thesis is finally concluded with Chapter five-Conclusions and Future scope, this chapter briefly states the conclusions drawn from the research and makes recommendations for future work based on the success and challenges from the present work. An Appendix of some of the tables produced from the research is provided to complete the write up.
2.LITERATURE REVIEW
Several works are reported in literature on Zeolites and hardness removed by adsorption. Extensive has been collected in the preparation of Fly Ash and hardness removed by various adsorbents. Works on hydrothermal treatment are dominant on testing domain, on isotherm study have been found in literature. The literature pertaining to Zeolite, adsorption and some other relevant works are presented in the following.
Suleyman A. Muyibi et al (1995)22., posed in his project that softening hard water by Moringaoleifera seeds. In this, the preliminary investigations into the possible use of Moringa oleifera seed suspension for the softening of hard water are presented. Four water sources: synthetic water (distilled water spiked with calcium chloride), naturally hard surface water and groundwater from two tube wells at different locations were used for the study. Modified laboratory jar test procedures for coagulation studies were used for the experimental runs. Water hardness from the sources varied from 300 up to 1000 mg/l as CaCO3. The mechanism for softening was found to be due to adsorption with the adsorption isotherm approximating to the Langmuir type, and conversion of soluble hardness-causing ions to insoluble products by precipitation reactions.
Ibrahim S. Al-Mutaz et al (2004)13., studied that the Silica removal during Lime Softening in Water Treatment Plant. In this Silica, SiO2, is typically found in well water supplies. Most of the silica found in well waters is result of dissolving silica-containing rock. Silica content in brackish water is generally in the range of 20 to 60 ppm. In Salbukh water treatment plant, silica concentration of raw water is about 30 ppm. High concentration of silica causes membrane fouling in the reverse osmosis units. During lime softening process calcium and magnesium are precipitated. Calcium is deposited as calcium carbonate while magnesium is deposited as magnesium hydroxide. Upon precipitation, magnesium hydroxide forms larger floc that more readily adsorbs or entraps silica particles in the water.
Sutarno et al (2004)25., posed in his project that Faujasite was hydrothermally synthesized from fly ash at 1000C in alkaline solution by reflux with various concentration of HCl and fusion with NaOH (weight ratio of NaOH/fly ash = 1.2) pretreatments.Thermal stability of faujasite from fly ash was tested at 400-900oC and was compared with commercial zeolite Y. The solid products were characterized by X-ray diffraction method, chemical analysis and nitrogen adsorption (BET). Results showed that synthesis of faujasite from fly ash via fusion pretreatment with NaOH has resulted faujasite selectively, however, the faujasite obtained still showed relatively low thermal stability due to its relatively low of Si/Al mol ratio.
Doungmanee Rungsuk et al (2006)6., goes with the Studies on Coal fly ash (CFA) was used to synthesize zeolite by fusion method. The synthesis conditions were optimized to obtain the product with high cation exchange capacity (CEC). The fusion temperature that resulted in the best CEC result was 450oC. The optimal fusion time was found to be 2 hours and this gave the highest CEC of 219.7 meq/100g. The zeolite product was characterized using various techniques, i.e. X-ray diffraction scanning electron microscopy, and the results revealed that the zeolite of this work was closest to the property of the X- type zeolite.
Tembhurkar. A.R et al (2006)26., goes with the Studies on Fluoride Removal Using Adsorption process. Batch adsorption studies were undertaken to assess the suitability of commercially available activated charcoal to remediate fluoride-contaminated water. The effects of some of the major parameters of adsorption, viz. pH, and dose of adsorbent, rate of stirring, contact time and initial adsorbate concentration on fluoride removal efficiency were studied and optimized. The optimum sorbent dose was found to be 2.0 g/100 mL, equilibrium was achieved in 120minutes and enhanced adsorption was obtained at pH 2. Maximum fluoride removal was observed to be 94% at optimum conditions. Freundlich as well as Langmuir isotherms were plotted and kinetic constants were determined.
Faizul Che Pa et al (2007)7., goes with the Studies on Fly ash (PFA) is a complex material produced after combustion in coal-fired power plants. About half of this fly ash is disposed as solid wastes. A possible alternative to disposal of the fly ash is the synthesis of zeolite. Zeolite Boggsite (Na37Ca74Al185Si775O192 7H2O) was synthesized from fly ash by hydrothermal treatment with NaOH solutions as identified by x-ray diffraction. The zeolite type and degree of crystallization were found to be dependent on the reaction conditions and mineralogy of the raw material, particularly in terms of the relative concentrations of SiO2 and Al2O3.
Balaji. R et al (2008)4., studied the removal of Iron from drinking / ground water by using agricultural waste as natural adsorbents. The sustainability of good health depends upon the purity of water. However groundwater may be exposed towards to contamination by various anthropogenic activities such as agricultural, domestic and industrial. Groundwater quality problem are typically associated with high level of iron concentration in West Mugapair, Chennai. The normal drinking water contains permissible limit of iron concentration 0.3mg/L but the West The parameters such as effect of pH, adsorbent dosage, contact time, initial concentration, etc., were studied. Maximum removal of total iron was observed in the pH range of 4-5. The highest percentage removal of total iron was observed at Coconut coir (96%) compared than sugarcane bagasse (93%).
Hung-Der Hsu et al (2008)12., studied the Silica pre-treatment for RO membrane b softening-adsorption. It states that previous literature has proven that both softening species of CaCO3(s) and Mg(OH)2(s) are capable of removing silica, but the mechanisms were not clearly described. Three different possible removal mechanisms were discussed here: Adsorption by aged CaCO3(s) and Mg(OH)2(s), Adsorption by amorphous (freshly precipitated) CaCO3(s) and Mg(OH)2(s) and Co-precipitation of CaCO3(s) and Mg(OH)2(s). A synthetic silica water was used to distinguish the above three mechanisms and the groundwater with 21 mgL-1 silica and high hardness was also used for comparison.
Winn-Jung Huang et al (2008)27., studied the effect of characteristics of activated carbon on removal of Bromate. This study evaluates how the characteristics of activated carbon (AC) influence the adsorption–reduction of Bromate (BrO3-) by performing kinetic and isotherm tests. Experimental results reveal that both physical and chemical effects simultaneously affect the adsorption–reduction process. The wood-based carbons contained more mesopores than coconut- and coal-based carbons, resulting in the adsorption of more BrO3-. The equilibrium and maximum adsorption capacities were calculated as a function of the effect of mesopore volume.
Gagik Badalians Gholikandi et al (2010)8., studied the natural Zeolites Application as sustainable adsorbent for heavy metals removal from drinking water. There are great resources of natural Zeolite in Iran. Zeolite, an inorganic ion-exchanger, may be used as a suitable technical-economical solution for water treatment in many regions of Iran. In this study, the characterization of natural Zeolite Natrolite and the feasibility of removing hardness, cations, and anions were investigated. First, zeolite composition and type were determined using X-ray Fluorescence (XRF), thermal analysis, and infrared spectrometer. However, by pH increases in the water sample, the arsenate anion removal arbitrarily changed: less in pH=3. But the removal efficiency got better in alkaline pH. It is obvious that by increasing the pH of water samples, the removal efficiency will decrease. In spite of that, the removal efficiency of chromate ion, in acidity pH, was more than Alkaline. The maximum removal of Cr occurred in acidic media at pH<1.5
Gamal O. El-Sayed (2010)9., studied the removal of water hardness by adsorption on Peanut hull. Studies on a batch sorption process using peanut hull as a low cost sorbent was investigated to remove calcium and magnesium ions from aqueous solutions. The influence of operational conditions such as contact time, metal ion initial concentration, sorbent mass, solution initial pH, agitation speed, ionic strength and temperature, on the sorption kinetics of calcium and magnesium were studied.
Anburaj Raj et al (2011)2., studied the Calcium removal from aqueous solution by marine Cyanocobacterium, Gloeocapsa species: adsorption kinetics and equilibrium studies. Calcium is an essential macronutrient required for healthy human life. It is abundantly present in seawater and its extraction is possible through biological means by using cyanobacteria. Efficiency of the marine cyanobacteria in removal of calcium is little known. Hence, the present work analysed adsorption kinetics of calcium from aqueous solution by using the marine cyanobacterium (Gloeocapsa sp.) in batch culture methods. The effect of initial calcium concentration, temperature, and adsorbent mass on calcium removal was tested. The process of calcium adsorption followed pseudo second-order rate expression and obeyed the Langmuir’s model with high correlation coefficient (R2>1). The results indicate that Gloeocapsa species is a potential adsorbent for calcium, as evident by its high calcium adsorption value under optimal culture conditions.
Jagdish Singh et al (2011)14., studied the dynamics of adsorption isotherms for the treatment conductivity and hardness of effluent with novel isolated microorganism. Microorganisms possess a capacity to accumulate organic and inorganic matter in their body, evoke the process of their enzymatic transformation and adsorb them on the cell surface. Detailed batch studies with the selected JR11 microbial biomass as a adsorbent, has been carried out to investigate the effect of pH, contact time on the adsorption isotherm for Hardness and Conductivity. Isolated bacterial strains JR11 posses the capacity to reduce Hardness and Conductivity of water about 80±2 % at pH 7 with contact time of 85 minutes. The adsorption kinetic study satisfied the model Langmuir and Freundlich equilibrium constant. Adsorption capacity was found to be 47.2 and 26 mg/g of adsorbent for Hardness and Conductivity respectively.
Bhaumik. R et al (2012)5., studied that the Eggshell powder as an adsorbent for removal of Fluoride from aqueous Solution: equilibrium, kinetic and thermodynamic Studies. He states that a new medium, eggshell powder has been developed for fluoride removal from aqueous solution. Fluoride adsorption was studied in a batch system where adsorption was found to be pH dependent with maximum removal efficiency at 6.0. The experimental data was more satisfactorily fitted with Langmuir isotherm model. The kinetics and the factor controlling adsorption process fully accepted by pseudo-second-order model were also discussed. Ea was found to be 45.98 kJmol-1 by using Arrhenius equation, indicating chemisorption nature of fluoride onto eggshell powder.
Pankaj Garg et al (2012)19., studied the Adsorption of Fluoride from drinking water on Magnesium substituted Hydroxyapatite. In the present study performance of synthesized adsorbent was investigated for fluoride removal from drinking water under batch mode operations. Magnesium substituted Hydroxyapatite (Mg-HAp) which is a calcium-magnesium based adsorbent was characterized using XRD. The effect of different parameters such as pH, initial fluoride concentration, contact time and co-existing ions (Bicarbonate, Sulphate, Chloride, Nitrate) were studied to understand the adsorption behaviour of sorbent under various conditions. Adsorption kinetics followed a typical kinetic curve and pseudo second order rate model described the kinetic sorption process indicating that chemical adsorption, rather than mass transfer, is the rate determining step. Equilibrium was reached in less than 24 hours of contact time and equilibrium data followed Freundlich isotherm model more closely as compared to Langmuir isotherm model.
Ahmed Mahdi Saeed et al (2013)1., studied the new approach for removal of total hardness (Ca2+, Mg2+) from water using commercial polyacrylic acid hydro gel beads, study and application. Adsorption and water treatment of Ca (II) and Mg (II) hardness were investigated via adsorption of metal ions onto commercial polyacrylic acid hydro gel beads as a novel sorbent for metal ions (Ca2+, Mg2+) removal and water treatment. Batch equilibrium technique was carried out under the influence of solution pH, contact time, sorbent dosage, initial metal concentration and competitive study. The maximum metal ions capacity (Ca2+, Mg2+) values were identified as 171.2 and 193.6, respectively. Sorption equilibrium was established in 24 hr and the data were described by both Langmuir and Freundlich models. The potential application of this sorbent for water treatment and metal ions removal from water samples is successfully accomplished.
Ashraful Islam et al (2013)3., studied the removal of pH, TDS (total dissolved solids) and Colour from Textile Effluent by Using Coagulants and Aquatic/Non Aquatic Plants as Adsorbents. The pH of Textile effluent is generally high because of use of many alkaline substances in Textile processing. The total dissolved solids (TDS) are those solids remain as soluble form in Textile effluent. There are several methods available for removal of TDS and colour from Textile effluent such as, ion exchange, coagulation and flocculation, biological decolourization, adsorption etc. Among all these methods adsorption is still a procedure of choice for TDS and colour removal. Several naturally occurring aquatic/non aquatic plants have been used in this work as adsorbents. These were water hyacinth, water lily and bark of plantain plant (banana).
Gandhi. N et al (2013)11., studied the adsorption studies of Chromium by using low cost adsorbents. The presence of toxic heavy metals such as Chromium contaminants in aqueous streams, arising from the discharge of untreated metal containing effluents into water bodies, is one of the most important environmental problems. Adsorption is one of the effective techniques for Chromium removal from wastewater. In the present study, adsorbent was prepared from low cost adsorbents and studies were carried out for Chromium removal. Batch adsorption studies demonstrated that the adsorbents had significant capacity to adsorb the Chromium from aqueous solution. It was found that the adsorption increased with increase in contact time and adsorbent dosage. An attempt was made to study mixed Algae as an adsorbent for removal of Chromium.
Mohammad Noori Sepehr (2013)17 et al., studied the removal of hardness agents, Calcium and Magnesium, by natural and Alkaline modified Pumice stones in single and binary systems. Natural and alkaline modified pumice stones were used for the adsorption of water hardening cations, Ca+2 and Mg+2. The adsorbents were characterized using XRF, XRD, SEM and FTIR instrumental techniques. At equilibrium time and for 150 mg/L of a given cation, removal efficiencies were 83% and 94% for Calcium and 48% and 73% for Magnesium for raw and modified Pumices, respectively. The optimal pH for raw and modified Pumices were found to be 6.0, leading to the removal of 79 and 96% of Calcium and 51 and 93% of Magnesium by 10 g/L of raw and modified Pumice adsorbents, respectively.
Patil. I.D et al (2013)20., studied the ground water nitrate removal by using ‘Chitosan’ as an adsorbent. Environmental pollution is the most terrible ecological crisis to which we are subjected today. Today the environment has become foul, contaminated, undesirable, and therefore harmful for the health of living organisms, including man. The most common contaminant identified in ground water is dissolved nitrogen in the form of Nitrate (NO3).Decomposition of organic matter present in soils leaching, of soluble fertilizers, human and animal excreta are the source of nitrate in subsurface waters. This research work is for to find preventive measures to avoid the nitrate pollution. This paper explains suitability of naturally available adsorbent i.e. ‘Chitosan’ for removal of nitrates from water is studied. The work is for the use of Chitosan in the different forms and concentrations to achieve the task.
Ravi Kumar. K et al (2013)21., studied the heavy metal removal from water using Moringa oleifera Seed coagulant and double filtration. The quality and accessibility of drinking water are of paramount importance to human health. Drinking water may contain disease-causing agents and toxic chemicals and to control the risks to public health, systematic water quality monitoring and surveillance are required. Thousands of chemicals have been identified in drinking water supplies around the world and are considered potentially hazardous to human health at relatively high concentrations. Heavy metals are the most harmful of the chemical pollutants and are of particular concern due to their toxicities to humans. Moringa oleifera seed acts as a natural coagulant, adsorbent and antimicrobial agent. It is believed that the seed is an organic natural polymer.
Satyanarayana. P et al (2013)22. studied the urban groundwater quality Assessment: A Case Study of Greater Visakhapatnam Municipal Corporation Area (GVMC), Andhra Pradesh, India. Water is indispensable for the existence and survival of life on earth. Water is needed in almost every sphere of human activity. Groundwater pollution is one of the environmental problems in urban areas. The paper presents urban groundwater quality assessment and analyzed for their physicochemical characteristics. The results of this analysis were compared with the water quality standards of WHO, BIS and CPHEEO. The water samples have been collected from 21 bore wells in GVMC area in Visakhapatnam city.
Kannan. D et al (2014)15., studied the removal of hardness (Ca2+, Mg2+) and Alkalinity from ground water by low cost adsorbent using Phyllanthus emblica wood. The main problem of the locality is the drinking water certain health problems are associated with people living in hills that are because of the presence of excess of ions and other impurities. The present study was conducted to analyze the some physicochemical parameters and removal of ions using Phyllanthus emblica adsorbent for purification of ground water for drinking purpose. In present study various dose of Phyllanthus emblica carbon are taken and checked for the efficiency dose on ground water. After treatment of water sample with Phyllanthus emblica carbon were analyzed for different parameters like TDS, Mg2+, Ca2+, Alkalinity, electrical conductivity all parameters were reduced with increased dose of Phyllanthus emblica carbon.
Khadse Shaila et al (2014)16., studied that the Synthesis of Zeolite using Fly ash and its application in Removal of Cu2+, Ni2+, Mn2+ from Paper Industry Effluent. In this paper An improved synthesis for fly ash based Zeolite has been attempted and studies have been carried out for the removal of Cu2+, Ni2+ and Mn2+ from paper industry wastewater. The parameters affecting adsorption process, such as initial pH, weight of Zeolite, contact time were investigated. The transition metal ions present in the waste Cu2+, Ni2+ and Mn2+ shows better adsorption capacities on synthesized zeolite. The order of removal of heavy metal ions is Ni2+ > Mn2+ > Cu2+.
Mohammad Almjadleh et al (2014)18., studied the use of natural and modified Jordanian Zeolitic tuff for removal of Cadmium from aqueous solutions. The possibility of using natural and modified Jordanian Zeolite for removal of Cadmium as a model component of heavy metals has been considered. Bench-scale tests using both aqueous solutions and real water under different experimental conditions have been employed. Different parameters, including metal ion concentration, modifier type, water quality and pH, have been studied. Synthetic Zeolite Molecular Sieve (4A) as control material has been also considered for the purpose of comparison. Hardness in water was found to highly impede adsorption. Sorption equilibrium was achieved within 250 min. The equilibrium isotherm data of Cadmium Zeolitic tuff were represented by Langmuir and Freundlich isotherm models, but they were well represented by Langmuir model.
Shailey Singhal et al (2014)23., studied the Bio-adsorbent: A cost-effective method for effluent treatment. Contamination of water with unwanted particles is a challenging problem for maintaining the quality and hygiene of water. Banana peels, the fruit waste were used to prepare environment benign bio-adsorbent for the adsorption of impurities from aqueous solution. They were washed, cut, dried and ground into powder of sieve size 150-212 μm before being used in the treatment of industrial water. Important parameters such as pH, colour, odour, alkalinity, hardness, residual free Chlorine, turbidity, total dissolved solids, chloride, fluoride, sulphate, metal ions, dissolved oxygen, biological Oxygen demand, chemical Oxygen demand and heavy metals were evaluated before and after the processing of water through bio adsorbent. Besides obtaining positive results in all parameters, bio adsorbent was found to be effective especially in the removal of iron and arsenic under specified conditions reaching a value of 30.45% and 25.12% respectively. The process provides a very low cost technique for one of the most challenging problem of world.
Gandhi. N et al (2014)10., studied the adsorption of Chromium from aqueous solution by using Multani mitti. The urbanization rate in India is very fast. It has increased from 10.84 to 28.5% up to 2001. Unregulated growth of urban area cause many problems. Due to increase in population, urbanization and industrialization many waste materials are entering into waste water streams and water streams causing deadful diseases. One of the most toxic chemical which is entering into the surface water is hexavalent ChromiumThe study is carried out with respect to contact time, concentration, dosage, effect of pH and temperature. The experimental data tested with adsorption isotherm and kinetics studies.
Among all of those researches mentioned above, some of them are expensive in adsorbents and processes and no one in them are research about the effectiveness of one adsorbent over other adsorbents which are obtained from different sources. So, it is cleared that we have to find a most cost effective adsorbent which is highly effects the removal of hardness from water.
3. METHODOLOGY
3.1. Methods:
The method used in this study is Adsorption method which is an hardness removal and less time taking method. The removal efficiencies are found out by using EDTA method.
3.2. Materials:
Material which is used majorly in the preparation of Laboratory Prepared Zeolite is Fly Ash and the chemicals used are Sodium Carbonate, Sodium Hydroxide, Sodium Silicate, Sodium Alignate and Lime.
For the efficiency comparison with the Laboratory prepared Zeolite, three commercial adsorbents are used in the study. They are Commercial Zeolite, Activated Carbon and Silica gel.
The major ingredient Fly ash which is used in the present adsorbent preparation is briefly described below:
Fly Ash:
Fly Ash is one of the residues generated in combustion and comprises the fine particles that rise with the flue gases. Ash which does not rise is termed bottom Ash. In an industrial context, Fly Ash is usually refers to ash produced during combustion of coal. Fly Ash is generally captured by Electrostatic precipitators or other particle filtration equipment’s before the flue gases reach the chimneys of coal-fired power plants, and together with bottom Ash removed from the bottom of the furnace is in the case jointly known as coal Ash. Depending upon the source and makeup of the coal being burned, the components of the Fly Ash vary considerably, but all Fly Ash includes substantial amounts of Silicon dioxide (SiO2), Al2O3 and Calcium Oxide(CaO), both being endemic ingredients in many coal-bearing rock strata.
Fly Ash particles are either solid or hollow and mostly glassy in nature. The particle size distribution of most bituminous coal Fly Ashes is generally similar to that of silt. Rapid cooling off from the molten state as it leaves the flame causes Fly Ash to be predominately non crystalline with minor amounts of crystalline constituents, such as Mullite, Quartz, Magnetite and Hematite.
Other constituents which may be present in high Calcium Fly Ash include, Anhydrite, Lime, Alkali Sulphate, Melilite, Nephiline, Sodalite, C3S and C2A. Fly Ash as a material is siliceous are Aluminous with pozolonic properties. It is refractory and Alkaline in nature. The shape, fineness, particle size distribution, density and composition of Fly Ash particles influence the properties of end use products. Fly Ash produced at different power plants or at the one plant with different coal sources may have different colors. Although there may be difference in the Fly Ash from one plant to another, day to day variations in the Fly Ash from a given power plant are usually quite predictable, provided plant operation and coal source remain constant. However, there can be a sustainable variation in Fly Ash obtained from burning coal with other fuels or with other combustible materials. As long as the basic operating parameter at the power plant does not change, Fly Ash from a known source supplied by a reputable Ash marketing organization should be a consistent, qualify controlled product.
The ways of Fly Ash utilization include (approximately in order of decreasing importance):
Ø Concrete production, as a substitute material for Portland cement and sand.
Ø Embankments and other structural fills(usually for road construction).
Ø Waste stabilization and solidification.
Ø Cement clinkers production-(as a substitute material for clay).
Ø Mine reclamation.
Ø Stabilization of soft soils.
Ø Road sub base construction.
Ø As aggregate substitute material (e.g. for brick production).
Ø Mineral filler in Asphaltic concrete.
Ø Agriculture uses soil amendment, fertilizer, cattle, feeders, soil stabilization in stock feed yards, agricultural stakes.
Ø Loose application on roads and parking lots for ice control.
More recently, Fly Ash has been used as a component in Zeolites.
All Chemicals and materials required for the EDTA test and adsorbtion method were taken from Vijayawada, Andhra Pradesh, India respectively. The materials are in powdered form with the fine size and used directly without any further grinding and sieving.
3.3. Adsorbents:
Synthetic hard water has prepared as instructed by Window on State Govt, where by 1g of cacl2 dissolved in a liter of deionized water to make water with hardness of 1000 mg/L as CaCl2 equivalent and this served as a stock solution. The initial concentrations are thus changed by varying the mix dosage of CaCl2.
3.4. Preparation of the Adsorbent LPZ:
Fly Ash contains Silica, Alumina with other impurities in small quantities. Analysis of Fly Ash sample used in the present study is shown in table 3.1 and the composition of Indian Fly Ash is shown in table 3.2.Silica and Alumina contained generally in the form of mixed Calcium and Aluminium Silicates.
Table 3.1 Chemical Composition of Fly Ash used in the present study
Compound | Composition of Fly Ash (%) |
SiO2 | 59.96 |
Al2O3 | 26.15 |
Fe2O3 | 5.85 |
Compound | Composition of Fly Ash (%) |
Silica(SiO2) | 49-69 |
Alumina(Al2O3) | 16-30 |
Iron Oxide(Fe2O3) | 4-10 |
Calcium Oxide(CaO) | 1-4 |
Magnesium Oxide(MgO) | 0.2-2 |
Sulphur | 0.1-2 |
Loss of Ignition | 0.5-3 |
Fly Ash and Sodium Hydro oxide (NaoH) is taken in ratios of , 10:90, 15:85, 20:80, 30:70 and kept in the furnace at different temperatures 300, 400, 500, 600, 700oC for heat treatment for 2hrs. Ions of Na+ replaced surface ions of Ca2+/Mg2+ at these temperatures.
Once the activation time was reached, the contents were cooled. The Zeolite material was then washed with distilled water until residual NaoH was fully removed. Thus the sample is filtered & dried in an oven at 100oC for 2hrs and its CEC is determined to check the Zeolite content.
The treated Fly Ash is made into pellets by agglomeration with Lime, Sodium Silicate and Sodium Alignate. Now the pellets are taken in bed and same procedure is carried out by running hard water through a column and collected water is analyzed for Zeolite CEC.
The Zeolitic sample tested for cation exchange capacity by the following method.
3.5. Testing of LPZ for CEC:
1g of Zeolite is taken in a Conical Flask and 20 mL of raw water is added and shaken thoroughly for 5 minutes in orbital shaker. The treated Zeolite is filtered and washed several times with DM water. The filtrate and washing are made into standard volume and estimated for total Ca2+, Mg2+ ions by EDTA method as obtained and noted as V1. Following the same procedure,25 mL of raw water is estimated for its Ca2+,Mg2+ ions content and noted as V2.
Difference of the above values represented m mole of Ca2+/kg of Zeolite. It is observed that raw Fly Ash contains 130 m mole kg-1 while for the present Zeolite ranges from 1560-7500 m mole kg-1 depending on the treatment it has undergone.
As Fly Ash contains mixed Silicates of Sodium and Aluminium but the exact values depend on the coal from Fly Ash have been generated and temperatures through which it has been passed. In the present study, Fly Ash and NaoH is taken in different temperatures 300, 400, 500, 600, 700oC. Part of NaoH attached to Fly Ash enhancing Si:Na ratio. Thus Fly Ash is expected to be of Sodium Alumino Silicate with replaceable charges. Exact structure elucidation is complex procedure but can be observed by measuring cation exchange capacity. Again the procedure is carried out until regenerated sample, CEC is determined, which is done in present study by the removal of hardness to water.
3.6. Determination of total hardness
Principle:
A water sample is buffered to pH 10 and taken to into a Conical Flask. If an indicator dye like EBT, when added to a solution containing Calcium and Magnesium ions, the colour the solution turns to wine red.
EDTA, the titrant, complexes the Magnesium and Calcium ions removing them from association with the indicator.
When all the ions of Ca and Mg are complexed with EDTA, the indicator will turn blue. This is the end point of the titration.
Apparatus required:
Ø Burette with Burette stand,
Ø Pipettes with elongated tips,
Ø Conical Flask(Erlenmeyer Flask),
Ø 250 mL graduated cylinders,
Ø Standard Flask,
Ø Wash bottle,
Ø Beaker.
Normal fiter paper
Chemicals required:
Ø Ammonium Chloride,
Ø Ammonium Hydroxide,
Ø EDTA(Disodium salt of EDTA),
Ø Erichrome Black T,
Ø Magnesium Sulphate.
Testing of water sample:
Ø Rinse and fill the burette with 0.02M EDTA solution and adjust it to zero, then fix it to Burette stand.
Ø Take out 20 mL of hard water sample into pipette and transfer it to a clean 250 mL Conical Flask.
Ø Add 2mL of Ammonia buffer solution to the water sample so that the pH will be maintained between 9 and 10.
Ø Add few drops of EBT indicator to the Conical Flask and the sample turns to wine red in colour.
Ø Titrate the sample against the EDTA solution in the Burette till all Calcium and Magnesium ions present the sample reacts with the EDTA. The appearance of blue colour indicates that Ca and Mg ions are complexed with EDTA and forms a metal EDTA complex that is the end point of the titration.
Ø Note down the burette reading.
Ø The value of the titration is noted in mL
Ø Repeat the titration for concordant value.
Finding the total hardness by using the following formula (Eq.1):
Total hardness = (V1*N*50*1000)/(V2) mg/L (1)
Here V1 is the volume of EDTA (mL),
V2 is the volume of sample taken (mL),
N is the normality of EDTA = 0.02N,
50 is the equivalent weight of CaCL2 (mg).
3.7. Batch experiments:
All experiments were conducted in batch mode in 250 mL conical flasks. Several operating parameters including adsorbent mass (1-15 g/L), initial ion concentrations (100-1500 mg/L) and contact time (1-15 minutes) were investigated. Optimized adsorption times for Commercial Zeolite, LPZ, Activated Carbon and Silica gel adsorbents were first examined by varying the contact time at room temperature for an adsorbent mass of 1g/L of solution. For this purpose, 1g of adsorbent were added to 1L of solution in a conical flask containing Ca+2 or Mg+2 cations at a concentration in the range of 1500 mg/L. The mixture was then shaken at 480 rpm. Samples were taken at predetermined time intervals, filtered by normal filter papers in order to reduce the filtration losses and the ion concentration was measured by a titration method.
After finding the optimum time and the optimum dosage, fix the optimum time, dosage values and find the removal efficiencies at various initial ion concentrations like 100, 200, 400, 700, 1000, 1200, 1500 mg/L respectively by the four adsorbents. The removal efficiency (RE) was determined as follows (Eq. 2):
Removal efficiency (%) = (Ci-Ce) / Ci*100 (2)
The quantity of adsorbed hardness on the adsorbate; i.e. uptake was calculated by the difference of initial and final (or) equilibrium concentrations following the equation (Eq. 3):
qe = (Ci-Ce)*V/m (3)
In the above equations:
Ci is the initial ion concentration (mg/L),
Ce is the equilibrium (or) final ion concentration (mg/L),
qe is the quantity of hardness adsorbed on the adsorbate (mg/g),
m is the amount of adsorbate (g),
V is the volume of the solution (L)
Several batch experiments were conducted to find the optimum time for the four adsorbents. By using the optimum time, we find the optimum dosage required by the four adsorbents, finally, we find the removal efficiency for various initial ion concentrations by the four adsorbents.
4. RESULTS AND DISCUSSIONS
The effects of three different factors on the adsorption were investigated. These factors included the amounts of adsorbent, contact time, initial concentration of water sample. The results evaluated are based on EDTA method.
The removal efficiency values are listed in the table, which are followed on the numbering. The relevant figure, which shows the graphical representation of the effect contact time, adsorbent dose, initial concentration on the hardness removal are also listed number wise.
The adsorption of hardness ions on to the adsorbent prepared in the laboratory and gives an efficiency comparison with commercially available adsorbents.
4.1. Effect of contact time on hardness removal:
A). Commercial Zeolite:
a) Data:
Initially the water sample has a concentration of 1500 mg/L, by using Commercial Zeolite at an initial dosage of 1 g/L, 2 % efficiency is obtained initially by the solution with 1min shaking by using orbital shaker. After 4 min shaking, I got 10.6% efficiency which is sufficient for the regular water treatment for residential, commercial or industrial use. But, to get the equilibrium state the adsorbent requires 5 min and efficiency observed is 11.6 %. The trial is continued up to 7 min to show that the adsorbent gets an equilibrium state, the removal efficiencies at various time intervals are shown in the following table 4.1, the relevant graph is drawn and shown in fig 4.1:
Table 4.1 Data for the effect of contact time on hardness removal by Commercial Zeolite at the initial ion concentration (Ci) is 1500 mg/L:
Contact Time, minutes | Ce, mg/L | Removal efficiency, % |
1 | 1470 | 2 |
2 | 1420 | 5.3 |
3 | 1360 | 9.3 |
4 | 1340 | 10.6 |
5 | 1325 | 11.6 |
6 | 1325 | 11.6 |
7 | 1325 | 11.6 |
The effect of the contact time was studied at 1, 2, 3, 4, 5, 6, 7 minutes respectively. Fig 4.1 shows the relationship between contact time and hardness removal efficiency. Observation reveals that the removal of hardness ions improved by increasing the contact time. The percentage hardness removal approached equilibrium within 5 minutes. Further increase in contact time does not show significant change in hardness. This might be due to fact that, large number of vacant surface sites is available for the adsorption during the initial stage and with the passage of time. After some times, repulsive forces between solute molecules on solid phase and liquid phase create difficultness for the solute molecules to occupy remaining vacant surface sites.
Fig 4.1 Effect of contact time on hardness removal by Commercial Zeolite: Hardness 1500 mg/L, Dose=1g/L.
B). Laboratory prepared Zeolite:
a) Data:
Initially the water sample has a concentration of 1500 mg/L, by using LPZ at an initial dosage of 1 g/L, 2 % efficiency is obtained initially by the solution with 1min shaking by using orbital shaker. After 5 min shaking, I got 13 % efficiency which is sufficient for the regular water treatment for residential, commercial or industrial use. But, to get the equilibrium state the adsorbent requires 6 min and efficiency observed is 17 %. The trial is continued up to 8 min to show that the adsorbent gets an equilibrium state, the removal efficiencies at various time intervals are shown in the following table 4.2, the relevant graph is drawn and is shown in fig 4.2:
Table 4.2 Data for the effect of contact time on hardness removal by LPZ:
Contact Time, minutes | Ce, mg/L | Removal efficiency, % |
1 | 1470 | 2 |
2 | 1400 | 7 |
3 | 1360 | 9 |
4 | 1320 | 12 |
5 | 1310 | 13 |
6 | 1250 | 17 |
7 | 1250 | 17 |
8 | 1250 | 17 |
The effect of the contact time was studied at 1, 2, 3, 4, 5, 6, 7, 8 minutes respectively. Fig 4.2 shows the relationship between contact time and hardness removal efficiency. Observation reveals that the removal of hardness ions improved by increasing the contact time. The percentage hardness removal approached equilibrium within 6 minutes. Further increase in contact time does not show significant change in hardness. This might be due to fact that, large number of vacant surface sites is available for the adsorption during the initial stage and with the passage of time. After some times, repulsive forces between solute molecules on solid phase and liquid phase create difficultness for the solute molecules to occupy remaining vacant surface sites.
Fig 4.2 Effect of contact time on hardness removal by Commercial LPZ: Hardness 1500 mg/L, Dose=1g/L.
C) Activated Carbon:
a) Data:
Initially the water sample has a concentration of 1500 mg/L, by using Activated Carbon at an initial dosage of 1 g/L, 4 % efficiency is obtained initially by the solution with 1min shaking by using orbital shaker. After 8 min shaking, I got 15.66% efficiency which is sufficient for the regular water treatment for residential, commercial or industrial use. Also, to get the equilibrium state the adsorbent requires 9 min and efficiency observed is 16.33%. The trial is continued up to 11 min to show that the adsorbent gets an equilibrium state, the removal efficiencies at various time intervals are shown in the following table 4.3, the relevant graph is drawn and is shown in fig 4.3:
Table 4.3 Data for the effect of contact time on hardness removal by Activated Carbon:
Contact Time, minutes | Ce, mg/L | Removal efficiency, % |
1 | 1440 | 4 |
2 | 1410 | 6 |
3 | 1395 | 7 |
4 | 1350 | 10 |
5 | 1330 | 11.33 |
6 | 1300 | 13.33 |
7 | 1280 | 14.66 |
8 | 1265 | 15.66 |
9 | 1255 | 16.33 |
10 | 1255 | 16.33 |
11 | 1255 | 16.33 |
b). Effect of contact time on hardness removal by Activated Carbon:
The effect of the contact time was studied at 1, 2, 3, 4, 5, 6, 7, 8, 9, 10, 11 minutes respectively. Fig 4.3 shows the relationship between contact time and hardness removal efficiency. Observation reveals that the removal of hardness ions improved by increasing the contact time. The percentage hardness removal approached equilibrium within 9 minutes. Further increase in contact time does not show significant change in hardness. This might be due to fact that, large number of vacant surface sites is available for the adsorption during the initial stage and with the passage of time. After some times, repulsive forces between solute molecules on solid phase and liquid phase create difficultness for the solute molecules to occupy remaining vacant surface sites.
Fig 4.3 Effect of contact time on hardness removal by Activated Carbon: Hardness 1500 mg/L, Dose=1g/L.
D). Silica gel:
a) Data:
Initially the water sample has a concentration of 1500 mg/L, by using Commercial Zeolite at an initial dosage of 1 g/L, 6% efficiency is obtained initially by the solution with 0.25 min shaking by using orbital shaker. After shaking0.5 min, I got 8% efficiency which is sufficient for the regular water treatment for residential, commercial or industrial use. But, to get the equilibrium state the adsorbent requires 0.5 min and efficiency observed is 8 %. The trial is continued up to 3 min to show that the adsorbent gets an equilibrium state, the removal efficiencies at various time intervals are shown in the following table 4.4,the relevant graph is drawn and shown in fig 4.4:
Table 4.4 Data for the effect of contact time on hardness removal by Silica gel:
Contact Time, minutes | Ce, mg/L | Removal efficiency, % |
0.25 | 1410 | 6 |
0.5 | 1380 | 8 |
1 | 1380 | 8 |
2 | 1380 | 8 |
3 | 1380 | 8 |
The effect of the contact time was studied at 0.25, 0.5, 1, 2, 3 minutes respectively. Fig 4.4 shows the relationship between contact time and hardness removal efficiency. Observation reveals that the removal of hardness ions improved by increasing the contact time. The percentage hardness removal approached equilibrium within 0.5 minutes. Further increase in contact time does not show significant change in hardness. This might be due to fact that, large number of vacant surface sites is available for the adsorption during the initial stage and with the passage of time. After some times, repulsive forces between solute molecules on solid phase and liquid phase create difficultness for the solute molecules to occupy remaining vacant surface sites.
Fig 4.4 Effect of contact time on hardness removal by Silica gel: Hardness 1500 mg/L, Dose=1g/L.
4.2.Effect of Adsorbent dose on hardness removal:
A). Commercial Zeolite:
a) Data:
Initially the water sample has a concentration of 1500 mg/L, by using Commercial Zeolite at contact time of 5 min, 11.66% efficiency is obtained initially by the solution with the dosage of 1g/L. To get the equilibrium state the adsorbent requires 4 g/L and efficiency observed is 14.66 %. The trial is continued up to a dosage of 8 g/L to show that the adsorbent gets an equilibrium state, the removal efficiencies at various adsorbent dosages are shown in the following table 4.5, and the relevant graph is drawn and shown in fig 4.5:
Table 4.5 Data for the effect of adsorbent dose on hardness removal by Commercial Zeolite:
Dosage, g/L | Ce, mg/L | Removal efficiency, % |
1 | 1325 | 11.6 |
2 | 1300 | 13.33 |
4 | 1280 | 14.66 |
6 | 1280 | 14.66 |
8 | 1280 | 14.66 |
The effect of the adsorbent dose on hardness removal is shown in Fig 4.5. Adsorbent dose was varied between 1-8 g/L and it was indicated that the removal efficiency of hardness improved by increasing the adsorbent dose up to 4 g/L where further dose increase yields negligible adsorption. Greater availability of exchangeable sites at higher concentration of adsorbent is the reason for the increase in hardness removal with the increase in adsorbent dose. According to Chakrabarthy and Sharma, after a certain dose of adsorbent, the maximum adsorption is attained and hence the amount of ions remains constant even with further addition of adsorbent. That’s why beyond 4 g/L, the adsorption found to be constant.
Fig 4.5 Effect of adsorbent dose on hardness removal by Commercial Zeolite: Hardness 1500 mg/L, Contact time=5minutes.
B). Laboratory Prepared Zeolite:
a) Data:
Initially the water sample has a concentration of 1500 mg/L, by using Laboratory prepared Zeolite at contact time of 6 min, 17% efficiency is obtained initially by the solution with the dosage of 1g/L. To get the equilibrium state the adsorbent requires 5 g/L and efficiency observed is 30%. The trial is continued up to a dosage of 7g/L to show that the adsorbent gets an equilibrium state, the removal efficiencies at various adsorbent dosages are shown in the following table 4.6, and the relevant graph is drawn and shown in fig 4.6:
Table 4.6 Data for the effect of adsorbent dose on hardness removal by LPZ:
Dosage, g/L | Ce, mg/L | Removal efficiency, % |
1 | 1250 | 17 |
2 | 1200 | 20 |
3 | 1150 | 23 |
4 | 1000 | 27 |
5 | 1050 | 30 |
6 | 1050 | 30 |
7 | 1050 | 30 |
The effect of the adsorbent dose on hardness removal is shown in Fig 4.6. Adsorbent 1-7 g/L and it was indicated that the removal efficiency of hardness improved by increasing the adsorbent dose up to 7 g/L where further dose increase yields negligible adsorption. Greater availability of exchangeable sites at higher concentration of adsorbent is the reason for the increase in hardness removal with the increase in adsorbent dose. According to Chakrabarthy and Sharma, after a certain dose of adsorbent, the maximum adsorption is attained and hence the amount of ions remains constant even with further addition of adsorbent. That’s why beyond 12 g/L, the adsorption found to be constant.
Fig 4.6 Effect of adsorbent dose on hardness removal by LPZ: Hardness 1500 mg/L, Contact time=6 minutes.
C). Activated Carbon:
a) Data:
Initially the water sample has a concentration of 1500 mg/L, by using Activated Carbon at contact time of 9 min, 16.33 % efficiency is obtained initially by the solution with the dosage of 1g/L. To get the equilibrium state the adsorbent requires 2 g/L and efficiency observed is 18.66 %. The trial is continued up to a dosage of 6 g/L to show that the adsorbent gets an equilibrium state, the removal efficiencies at various adsorbent dosages are shown in the following table 4.7, and the relevant graph is drawn and shown in fig 4.7:
Table 4.7 Data for the effect of adsorbent dose on hardness removal by Activated Carbon:
Dosage, g/L | Ce, mg/L | Removal efficiency, % |
1 | 1255 | 16.33 |
2 | 1220 | 18.66 |
4 | 1220 | 18.66 |
6 | 1220 | 18.66 |
The effect of the adsorbent dose on hardness removal is shown in Fig 4.7 Adsorbent dose was varied between 2-10 g/L and it was indicated that the removal efficiency of hardness improved by increasing the adsorbent dose up to 4 g/L where further dose increase yields negligible adsorption. Greater availability of exchangeable sites at higher concentration of adsorbent is the reason for the increase in hardness removal with the increase in adsorbent dose. According to Chakrabarthy and Sharma, after a certain dose of adsorbent, the maximum adsorption is attained and hence the amount of ions remains constant even with further addition of adsorbent. That’s why beyond 4 g/L, the adsorption found to be constant.
Fig 4.7 Effect of adsorbent dose on hardness removal by Activated Carbon: Hardness 1500 mg/L, Contact time=9 minutes.
D). Silica gel:
a) Data:
Initially the water sample has a concentration of 1500 mg/L, by using Silica gel at contact time of 0.5 min, 8% efficiency is obtained initially by the solution with the dosage of 1g/L. To get the equilibrium state the adsorbent requires 4 g/L and efficiency observed is 12%. The trial is continued up to a dosage of 8 g/L to show that the adsorbent gets an equilibrium state, the removal efficiencies at various adsorbent dosages are shown in the following table 4.8, and the relevant graph is drawn and shown in fig 4.8:
Table 4.8 Data for the effect of adsorbent dose on hardness removal by Silica gel:
Dosage, g/L | Ce, mg/L | Removal efficiency, % |
1 | 1380 | 8 |
2 | 1350 | 10 |
4 | 1320 | 12 |
6 | 1320 | 12 |
8 | 1320 | 12 |
The effect of the adsorbent dose on hardness removal is shown in Fig 4.8 Adsorbent dose was varied between 1-8 g/L and it was indicated that the removal efficiency of hardness improved by increasing the adsorbent dose up to 4 g/L where further dose increase yields negligible adsorption. Greater availability of exchangeable sites at higher concentration of adsorbent is the reason for the increase in hardness removal with the increase in adsorbent dose. According to Chakrabarthy and Sharma, after a certain dose of adsorbent, the maximum adsorption is attained and hence the amount of ions remains constant even with further addition of adsorbent. That’s why beyond 4 g/L, the adsorption found to be constant.
Fig 4.8 Effect of adsorbent dose on hardness removal by Silica gel: Hardness 1500 mg/L, Contact time=0.5 minutes.
4.3. Effect of Initial ion concentration on hardness removal:
A). Commercial Zeolite:
a) Data:
By using Commercial Zeolite at a dosage of 4g/L with an optimum contact time of 5 min, the removal efficiencies are decreased with increasing the initial ion concentrations. The percentages of removal efficiencies at various initial ion concentrations are shown in the following table 4.9,the relevant graph is drawn and shown in fig 4.9
Table 4.9 Data for the effect of initial ion concentration on hardness removal by Commercial Zeolite:
Ci, mg/L | Ce, mg/L | Removal efficiency, % |
100 | 80 | 20 |
200 | 170 | 15 |
400 | 360 | 10 |
700 | 640 | 8.5 |
1000 | 940 | 6 |
1200 | 1140 | 5 |
1500 | 1440 | 4 |
The effect of the Initial ion concentration on hardness removal is shown in Fig 4.9. The initial ion concentration was varied between 100-1500 mg/L and it was indicated that the removal efficiency decreases by increasing the initial ion concentration.
Fig 4.9 Effect of initial ion concentration on hardness removal by Commercial Zeolite: Contact time=5 minutes, Dosage=4g/L.
B). Laboratory Prepared Zeolite:
a) Data:
By using Laboratory prepared Zeolite at a dosage of 5 g/L with an optimum contact time of 6 min, the removal efficiencies are decreased with increasing the initial ion concentrations. The percentages of removal efficiencies at various initial ion concentrations are shown in the following table 4.10,the relevant graph is drawn and shown in fig 4.10:
Table 4.10 Data for the effect of initial ion concentration on hardness removal by LPZ:
Ci, mg/L | Ce, mg/L | Removal efficiency, % |
100 | 35 | 65.00 |
200 | 75 | 62.50 |
400 | 130 | 56.67 |
700 | 225 | 50.00 |
1000 | 330 | 45.00 |
1200 | 495 | 38.13 |
1500 | 685 | 31.50 |
The effect of the Initial ion concentration on hardness removal is shown in Fig 4.10. The initial ion concentration was varied between 100-1500 mg/L and it was indicated that the removal efficiency decreases by increasing the initial ion concentration.
Fig 4.10 Effect of initial ion concentration on hardness removal by LPZ: Contact time=6 minutes, Dosage=5g/L.
C). Activated Carbon:
a) Data:
By using Commercial Zeolite at a dosage of 2g/L with an optimum contact time of 9 min, the removal efficiencies are decreased with increasing the initial ion concentrations. The percentages of removal efficiencies at various initial ion concentrations are shown in the following table 4.11,the relevant graph is drawn and shown in fig 4.11:
Table 4.11 Data for the effect of initial ion concentration on hardness removal by Activated Carbon:
Ci, mg/L | Ce, mg/L | Removal efficiency, % |
100 | 90 | 10 |
200 | 170 | 15 |
400 | 350 | 12.5 |
700 | 640 | 8.5 |
1000 | 940 | 6 |
1200 | 1140 | 5 |
1500 | 1440 | 4 |
The effect of the Initial ion concentration on hardness removal is shown in Fig 4.11. The initial ion concentration was varied between 100-1500 mg/L and it was indicated that the removal efficiency decreases by increasing the initial ion concentration.
Fig 4.11 Effect of initial ion concentration on hardness removal by Activated Carbon: Contact time=9 minutes, Dosage=2g/L.
D). Silica gel:
a) Data:
By using Silica gel at a dosage of 4g/L with an optimum contact time of 0.5 min, the removal efficiencies are decreased with increasing the initial ion concentrations. The percentages of removal efficiencies at various initial ion concentrations are shown in the following table 4.12,the relevant graph is drawn and shown in fig 4.12:
Table 4.12 Data for the effect of initial ion concentration on hardness removal by Silica gel:
Ci, mg/L | Ce, mg/L | Removal efficiency, % |
100 | 90 | 10 |
200 | 180 | 10 |
400 | 370 | 7.5 |
700 | 640 | 8.5 |
1000 | 950 | 5 |
1200 | 1150 | 4.1 |
1500 | 1440 | 3 |
The effect of the Initial ion concentration on hardness removal is shown in Fig 4.12. The initial ion concentration was varied between 100-1500 mg/L and it was indicated that the removal efficiency decreases by increasing the initial ion concentration.
Fig 4.12 Effect of initial ion concentration on hardness removal by Silica gel: Contact time=0.5 minutes, Dosage=4g/L.
4.4. Comparison of four adsorbents by their equilibrium time and dose:
The values of equilibrium times and dosages of commercial Zeolite, LPZ, Activated Carbon and Silica gel are given below; here the contact time observed from 1-10 minutes, dosages are observed from 1-15 g/L.
Table 4.13 Data for the four adsorbents by their equilibrium time and dose:
Adsorbent | Optimum time (minutes) | Optimum dosage(g/L) |
Commercial Zeolite | 5 | 4 |
LPZ | 6 | 5 |
Activated Carbon | 9 | 2 |
Silica gel | 0.5 | 4 |
4.5. Maximum percentages of removal efficiencies of four adsorbents when treated for Contact time, Dosage, Initial ion concentration.
A). Contact Time:
When the adsorbents are treated for contact time, the maximum removal efficiency percentages are listed in the table 4.14 and relevant graph is drawn and is shown in fig 4.14:
Table 4.14 Data for the maximum removal efficiencies of four adsorbents when treated for contact time:
Adsorbent | Removal Efficiency |
Commercial Zeolite | 11.6 |
LPZ | 10 |
Activated Carbon | 16.33 |
Silica gel | 8 |
B). Adsorbent dosage:
When the adsorbents are treated for adsorbent dosage, the maximum removal efficiency percentages are listed in the table 4.15 and relevant graph is drawn and is shown in fig 4.15:
Table 4.15 Data for the maximum removal efficiencies of four adsorbents when treated for adsorbent dosage:
Adsorbent | Removal Efficiency |
Commercial Zeolite | 14.66 |
LPZ | 13 |
Activated Carbon | 18.66 |
Silica gel | 12 |
Fig 4.15 maximum percentages of removal efficiencies of four adsorbents, when treated for adsorbent dosage.
C). Initial ion concentration:
When the adsorbents are treated for initial ion concentration, the maximum removal efficiency percentages are listed in the table 4.16 and relevant graph is drawn and is shown in fig 4.16:
Table 4.16 Data for the maximum removal efficiencies of four adsorbents when treated for initial ion concentration:
Adsorbent | Removal Efficiency |
Commercial Zeolite | |
LPZ | |
Activated Carbon | |
Silica gel |
Fig 4.16 maximum percentages of removal efficiencies of four adsorbents when treated for Initial ion concentration.
5. CONCLUSIONS AND FUTURE SCOPE
5.1. Conclusions:
The main conclusions of the study are given below:
· The maximum hardness is removed by the Laboratory prepared Zeolite at the initial ion concentration 100mg/L .i.e, %.
· Activated carbon is highly efficient adsorbent at the higher concentration levels like 1500mg/L and the removal efficiency is 16.33%.
· The optimum adsorbent doses are 6, 12, 4 and 6 g/L, & contact times are 60, 30, 150 and 90 minutes for Commercial Zeolite, Laboratory prepared Zeolite, Activated carbon and Silica gel respectively.
· The laboratory prepared Zeolite is effective up to the concentration level of 500mg/L i.e., usable for drinking water.
5.2. Future scope:
· LPZ is effective up to 3 washes only, so, it is essential to improvise the capacity for more washes, hence to reduce the economy and for saving time.
· The continuation studies on this thesis to be concentrates on pH change, temperature variation and particle size.
APPENDIX
Sample calculation 1:
The hardness of the required sample is calculating by the following:
Determination of total hardness of the prepared stock solution:
Table 4.27 Data for finding hardness for the prepared stock solution:
S.No | Volume of the sample (mL) | Burette Reading (mL) | Volume of EDTA (mL) | |
Initial | Final | |||
1 | 20 | 0 | 9.9 | 9.9 |
2 | 20 | 0 | 10.2 | 10.2 |
3 | 20 | 0 | 10.2 | 10.2 |
Volume of EDTA = 10.2 mL
Normality of EDTA = 0.02N
Volume of the sample =20 mL
Equivalent weight of CaCO3 = 50 mg
Total Hardness = (Volume of EDTA*N*50*1000)/Volume of the sample
Calcium hardness as CaCO3 equivalent= (10.2*0.02*50*1000)/20
= 510 mg/L as CaCO3 equivalent
The total hardness of the sample = 510 mg/L
Sample calculation 2:
Determination of adsorption capacity (qe):
The adsorption capacity of a sample with an initial ion concentration of 100 mg/L as shown below:
The required formula is
qe = (Ci-Ce)*V/m
Here, Ci = 100 mg/L
Ce = 40 mg/L
V= 1 L
M = 2g
Therefore qe = (100-40)*1/2
=60/2
=30mg/g
5. CONCLUSIONS AND FUTURE SCOPE
5.1. Conclusions:
The main conclusions of the study are given below:
· The maximum hardness is removed by the Laboratory prepared Zeolite at the initial ion concentration 100mg/L .i.e, %.
· Activated carbon is highly efficient adsorbent at the higher concentration levels like 1500mg/L and the removal efficiency is 16.33%.
· The optimum adsorbent doses are 6, 12, 4 and 6 g/L, & contact times are 60, 30, 150 and 90 minutes for Commercial Zeolite, Laboratory prepared Zeolite, Activated carbon and Silica gel respectively.
· The laboratory prepared Zeolite is effective up to the concentration level of 500mg/L i.e., usable for drinking water.
5.2. Future scope:
· LPZ is effective up to 3 washes only, so, it is essential to improvise the capacity for more washes, hence to reduce the economy and for saving time.
· The continuation studies on this thesis to be concentrates on pH change, temperature variation and particle size
ABOUT THE AUTHOR
Having Natural Powers of Seeing Eating Smelling Hearing Talking Walking ***King
>
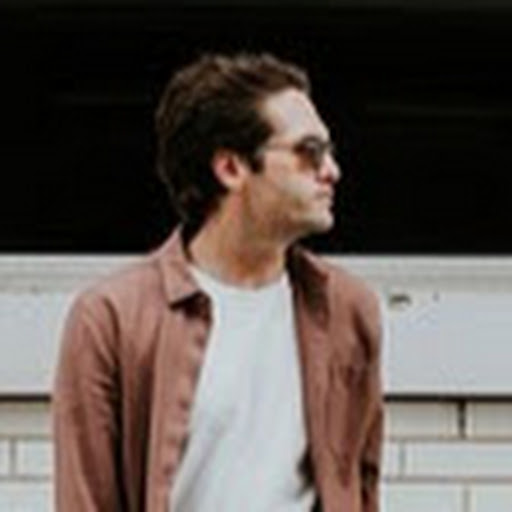
Having Natural Powers of Seeing Eating Smelling Hearing Talking Walking ***King
0 comments:
Post a Comment